
Radix 1.31 serial key or number

Radix 1.31 serial key or number
Effects of Radix Astragali and Its Split Components on Gene Expression Profiles Related to Water Metabolism in Rats with the Dampness Stagnancy due to Spleen Deficiency Syndrome
Abstract
Radix Astragali (RA) with slight sweet and warm property is a significant “qi tonifying” herb; it is indicated for the syndrome of dampness stagnancy due to spleen deficiency (DSSD). The purpose of this research was to explore effects of RA and its split components on gene expression profiles related to water metabolism in rats with the DSSD syndrome for identifying components representing property and flavor of RA. The results indicated that RA and its split components, especially polysaccharides component, significantly increased the body weight and the urine volume and decreased the water load index of model rats. Our data also indicated differentially expressed genes (DEGs) related to water metabolism involved secretion, ion transport, water homeostasis, regulation of body fluid levels, and water channel activity; the expression of AQP1, AQP3, AQP4, AQP5, AQP6, and AQP8 was improved; calcium, cAMP, MAPK, PPAR, AMPK, and PI3K-Akt signaling pathway may be related to water metabolism. In general, results indicate that RA and its split components could promote water metabolism in rats with the DSSD syndrome via regulating the expression of AQPs, which reflected sweet-warm properties of RA. Effects of the polysaccharides component are better than others.
1. Introduction
In traditional Chinese medicine (TCM), Chinese herbal medicines have four properties and five flavors, and various properties and flavors of herbs exert different effects. The action of a herbal medicine is always not one but many [1]. The property and flavor theory is a guideline for using the herbal medicines. The property of a Chinese medicinal herb affects material and energy metabolism. In addition, the material basis of the property and flavor of a herbal medicine can be split [2]. So far, however, there has been little scientific connotation of this theory based on the research, and we could not clearly explain its mechanism of property and flavor with modern medical theory. In this study, we tried to explore split components representing property and flavor of RA through studying effects of RA and its split components on gene expression profiles related to water metabolism in rats with the DSSD syndrome based on the property and flavor theory.
RA with slightly sweet flavor and warm property, known as Huang Qi in China, is one of the important “qi tonifying” or adaptogenic herbs. It possesses tonic, diuretic, and hepatoprotective properties and has been shown to exhibit immunomodulating, antioxidant, and antihyperglycemic effects [3–5]. The main components of RA include flavonoids, saponins, polysaccharides, amino acids, and some trance elements [4]. The China Pharmacopoeia recorded that RA affects the spleen and lung meridians and has the function of tonifying spleen qi [6]. It is indicated for symptoms of spleen qi deficiency such as fatigue, diarrhea, anemia, and loss of appetite [5].
Spleen governs dampness in the TCM theory. Spleen is the hub of water metabolism [7]. Water and cereal essence were digested, absorbed, and transported with the action of spleen qi. TCM holds the truth that the fat food, overfatigue, excessive thinking, and innate weakness of the body for a long time are the main causes of spleen qi deficiency. It has been confirmed that spleen qi deficiency will result in stagnancy of water and dampness [8, 9]. This is the pathogenesis of the DSSD syndrome. The clinical manifestations of DSSD include poor appetite, a bitter taste in the mouth, thick and slimy tongue, abdominal fullness, a feeling of discomfort, dizziness, weak or fatigued limbs, and frequently edema. Therefore, tonifying spleen qi and promoting diuresis are the main method for treating the DSSD syndrome. The DSSD syndrome is very common. Many chronic diseases such as obesity, ulcerative colitis, diabetes, and tumors may be accompanied with the DSSD syndrome, which will make disease recurrent and persistent [10–12]. However, there is no notion about the DSSD syndrome in modern medicine and there are no good treatments.
The effects of Chinese medicinal herbs on the syndrome involve multiple channels and targets because they contain complicated ingredients. The gene chip technology has a characteristic of high throughput, which can be used to analyze the complexity of herbs [13–16]. Therefore, in this study, we extracted the components of RA and observed effects of single components on water metabolism in rats with the DSSD syndrome to explore the mechanism and identify the bioactive components representing sweet-warm properties of RA using pharmacological experiment and gene chip technology.
2. Materials and Methods
2.1. Materials
RA (number 130107), the dried root of Astragalus membranaceus (Fisch.) Bge. var. mongholicus (Bge.) Hsiao, was purchased from Gansu province of China. Rat Gene Expression Microarray (8 × 60K, design ID: 028279), Gene Expression Hybridization Kit, the Quick Amp Labeling Kit, One-Color, Gene Chip Hybridization Oven, and Microarray Scanner were provided by Agilent Technologies Co., Ltd. (Shanghai, China); mirVana™ RNA Isolation Kit and 7500 Fast Dx Real-Time PCR Instrument were obtained from Applied Biosystems (Foster City, CA, USA); RNeasy Mini Kit was obtained from Qiagen Co., Ltd. (Hilden, Germany).
2.2. Animal
Fifty-six specific-pathogen-free (SPF), 4-week-old Wistar rats (half male and half female), weighing g, were purchased from Beijing Vital River Laboratory Animal Technology Co. Ltd. (Beijing, China). The animal certification number was SCXK (Jing) 2012-0001.
2.3. Animal Model
Rats were acclimated for 3 days in our animal facility and then randomly divided into 7 groups, a normal control group, a model group, a water decoction group, a flavonoids group, a saponins group, a polysaccharides group, and a residual aqueous solution group, with 8 rats in each group. Model rats with DSSD were induced according to the relevant reference [17]. Rats of the model group and the drug group were fed a special high-fat and low-protein diet improved from the AIN-76A purified rat diet and subjected to exhaustive swimming with tail load (10% of the body weight) every afternoon for 6 weeks. Rats were considered to be exhausted when their nose tip was submerged in water for 10 s. Rats of the normal control group were fed AIN-76A purified rat diet and distilled water.
2.4. Preparation of RA and Its Split Components
2.4.1. Extracts of RA and Its Split Components
RA was decocted and concentrated to 0.54 g (crude drug)/mL. RA was treated through water boiling and precipitation with ethanol. The precipitate was polysaccharides. The supernatant was extracted by ethyl acetate and n-butyl alcohol. Flavonoids and saponins were obtained, respectively. Flavonoids contained calycosin glycosides, 4.88 mg·g−1, ononin, 3.80 mg·g−1, calycosin, 7.78 mg·g−1, and formononetin, 4.79 mg·g−1. Saponins contained astragaloside, 4.40 mg·g−1, astragalus saponin I, 0.28 mg·g−1, astragalus saponin II, 1.42 mg·g−1, and astragalus saponin III, 1.31 mg·g−1. Polysaccharides component was 402.2 mg·g−1 crude polysaccharides.
2.4.2. Verification of Overlap among RA Split Components
The identification of overlap among RA split components was processed through similarity evaluation system for chromatographic fingerprint of TCM (Version 2012) with flavonoids as the control sample and principal component analysis using high-performance liquid chromatography-diode array detection (HPLC-DAD) data.
2.5. Experimental Protocol
After establishment of model rats, RA and its split components were administrated to rats. The dosage was determined from the product of highest daily dose (60 g/70 kg) of the Chinese Pharmacopoeia (2010 edition) and the equivalent dose coefficient. RA water decoction was given 5.40 g·kg−1·d−1. The dosage of the split components was the same as the content in the 5.40 g crude RA, such as the flavonoids component, 0.07 g·kg−1·d−1, the saponins component, 0.27 g·kg−1·d−1, the polysaccharides component, 1.41 g·kg−1·d−1, and the residual aqueous solution, 1.32 g·kg−1·d−1. The normal control group and the model group were given equal amounts of normal saline. Drugs were administered by gavage once a day for 14 days, 1 mL/100 g. After the last administration, rats were decapitated with their duodenum tissues separated at 4°C and put into liquefied nitrogen for storage.
2.6. Measurement of the Body Weight
After the last administration, the body weight of rats in each group was measured by electronic balance.
2.7. Measurement of the Water Load Index and the Urine Volume
The body weight of rats was detected after fasting for 12 hours, as a control value. Then rats were intraperitoneally injected with normal saline in the volume equal to 10% of the body weight. After that, rats were put into the metabolic cage, and the body weight was measured after 0, 1, 2, 4, and 6 hours. During this time, food and water were banned. Urine in 6 hours was collected. Ratio of the body weight loss (%) = (the body weight after water load − body weight before water load)/body weight before water load × 100. Water load chart between ratio of the body weight loss and time was drawn using origin 9.0; water load index was area under the curve of 6-hour water load.
2.8. Statistical Analysis
SPSS 21.0 was used for statistical analysis. The values for the body weight, water load index, and urine volume were all presented as the mean ± standard deviation (±SD). Differences between groups were tested by one-way ANOVA followed by LSD method to test for difference between the mean values of all groups. was considered statistically significant. The area under the curve of 6-hour water load was calculated using Origin 9.0.
2.9. Gene Chip Analysis
Total RNA was extracted using mirVana RNA Isolation Kit and purified with RNeasy Mini Kit according to the manufacturers’ instructions. Quality and concentration of RNA were checked by ND-2000 spectrophotometer. Equal amount of purified RNA from three different samples was mixed. Samples were hybridized separately into seven Agilent Rat Gene Expression Microarrays. 200 ng total RNA was used to synthesize double-stranded cDNA and then to produce biotin-tagged cRNA using the Quick Amp Labeling Kit, One-Color. The cRNA was purified by the RNeasy Mini Kit. The labeled cRNA samples were fragmented for 30 min at 60°C in the dark. The fragmented cRNA was hybridized to Agilent 8 × 60K Rat Gene Expression Microarray. Hybridization was performed using the Agilent Gene Expression Hybridization Kit at 65°C with rotation for 17 hours on an Agilent Gene Chip Hybridization Oven. The gene chips were washed followed by scanning with an Agilent Microarray Scanner. The images were converted into gene expression data by Agilent Feature Extraction software (version 10.7.1.1).
2.10. Functional and Pathway Enrichment Analysis of DEGs
Raw data obtained from gene chip test were imported into Agilent GeneSpring software (version 12.5) and normalized using quantile algorithm. The normalized values were compared between the model group and the normal control group and between treatment groups and the model group. The DEGs were screened using criterion of more than or less than 2-fold change. DAVID (https://david.ncifcrf.gov/) is an online program that provides a set of high-throughput gene functional annotation to understand the biological meaning behind plenty of genes [18]. To gain insight into the potential functional consequences and pathway changes induced by RA and its split components, GO and KEGG pathway enrichment analysis of DEGs were performed using DAVID database. The significant enrichment was defined as .
2.11. Quantitative Real-Time PCR Analysis
Two-step quantitative real-time PCR (qRT-PCR) was performed to confirm gene chip results according to Applied Biosystems’ guidelines. Two candidate genes were chosen for qRT-PCR according to the expression ratio in each group. The primers of candidate genes were designed using Primer Premier 5.0 software. Sequences of oligonucleotide primer pairs for selected genes were shown in Table 1. Amplification conditions were 95°C for 30 s, followed by 40 cycles of 95°C for 3 s, 60°C for 30 s, and 60°C for 30 s. Melting curve analysis was performed at the end of each PCR reaction to confirm if a single PCR product was detected. The housekeeping gene, glyceraldehyde-3-phosphate dehydrogenase (GAPDH), was used to normalize quantification of the mRNA targets. All the reactions were performed in triplicate. The method was used to calculate the relative gene expression levels.
|
3. Results
3.1. Identification of Overlap among RA Split Components
Four main components, polysaccharides, flavonoids, saponins, and residual aqueous solution constituents, were extracted from RA. The results of fingerprint showed that overlap among the four components was very low, which totally meets the requirement for experimental standard (Figure 1(a)). HPLC-DAD data was imported into SIMCA-P to process multivariate statistical analysis. Two-dimensional distribution of four split components is shown in Figure 1(b), which also indicated that the overlap was very low.
(a)
(b)
3.2. Comparisons of the Body Weight
Compared with the model group, the body weights of rats in the RA decoction group and split components groups were higher than that in the model group ( and , Figure 2(a)).
(a)
(b)
(c)
|
Influence of Plant Growth Retardants on Quality of Codonopsis Radix.
2. Results and Discussion
2.1. PGR Treatment of C. Radix Did Not Affect Lobetyolin and Atractylenolide III Contents
The contents of lobetyolin and atractylenolide III in the C. Radix samples were calculated by comparison with authentic standards. The average contents of lobetyolin in control and PGR-treated samples were 0.29 and 0.22 mg/g, respectively, and those of atractylenolide III were 0.017 and 0.019 mg/g, respectively. Neither compounds showed significant differences between the treated and the control groups (Figure 1).

Effect of plant growth retardant (PGR) on lobetyolin and atractylenolide III contents in C. Radix samples. (A) Chromatogram comparison of lobetyolin standard, and control and treatment groups. Lobetyolin (std.), lobetyolin standard (20 ng/μL, m/z = 397.1862, RT = 10.43 min); control, untreated C. Radix sample; treatment, PGR-treated C. Radix sample. (B) Chromatogram comparison of atractylenolide III standard, control and treatment groups. Atractylenolide III (std.), atractylenolide III standard (10 ng/μL, m/z = 249.1491, RT = 24.68 min). (C) & (D) Lobetyolin and atractylenolide III contents in control and treatment groups (as calculated from peak areas of samples and the standard).
There are two crucial active ingredients in C. Radix; lobetyolin and atractylenolide III. Lobetylin has been shown to have antioxidant [10], anti-inflammatory, immunity-enhancing, and stomach-protective activities, while atractylenolide III has been shown to have anti-inflammatory [11], stomach-protective [12], and anti-lung-cancer activities [13]. Since these two compounds contribute to the efficacy of C. Radix, many studies have analyzed changes in their abundance under various conditions or treatments, for example, sulfur fumigation, drying methods, and so on [14,15]. The results of the present study showed that these compounds showed only slight fluctuations after PGR treatment, indicating that PGR did not affect their biosynthetic pathways.
2.2. PGR Treatment Significantly Decreased Polysaccharides and Internal Volatiles Contents
The total polysaccharides content in PGR-treated samples (376.09 mg/g) was lower than that in untreated samples (542.50 mg/g; Figure 2). Among the 39 volatile compounds detected and identified in the GC-MS analysis, 14 showed a decreased abundance in the PGR-treated samples, while the others were unaffected by PGR treatment. The 14 compounds are shown in Table 1. Diisobutyl phthalate and methyl hexadecanoate contribute to the special flavor of C. Radix [6].

Effect of PGR on polysaccharides in C. Radix samples. Control, untreated C. Radix sample; treatment, PGR-treated C. Radix sample. The * indicates significant difference (p ≤ 0.05).
Table 1
Effect of PGR on internal volatiles in C. Radix samples.
Internal Volatile Metabolite | Treatment | Control | T/CK |
---|---|---|---|
Dodecane | 0.05 ± 0.01 | 0.09 ± 0.01 | 0.56 * |
Hexadecane | 0.13 ± 0.01 | 0.23 ± 0.02 | 0.57 * |
2-Furanmethanol | 0.06 ± 0.01 | 0.13 ± 0.01 | 0.46 * |
Phytane | 0.10 ± 0.01 | 0.17 ± 0.03 | 0.59 * |
Octacosane | 0.05 ± 0.01 | 0.10 ± 0.01 | 0.50 * |
Dihydroanethole | 0.27 ± 0.10 | 1.42 ± 0.28 | 0.19 * |
Oxalic acid, dodecyl 2-methylphenyl ester | 0.05 ± 0.01 | 0.11 ± 0.01 | 0.46 * |
Ethylhexyl benzoate | 0.04 ± 0.01 | 0.07 ± 0.00 | 0.57 * |
Methyl hexadecanoate | 0.16 ± 0.01 | 0.24 ± 0.03 | 0.67 * |
Diethyl Phthalate | 0.10 ± 0.01 | 0.14 ± 0.01 | 0.71 * |
n-Heptadecylcyclohexane | 0.03 ± 0.00 | 0.06 ± 0.01 | 0.50 * |
Methyl octadeca-9,12-dienoate | 0.11 ± 0.01 | 0.21 ± 0.04 | 0.52 * |
Diisobutyl phthalate | 0.72 ± 0.08 | 1.10 ± 0.10 | 0.66 * |
Phthalic acid, decyl 2,7-dimethyloct-7-en-5-yn-4-yl ester | 0.54 ± 0.07 | 0.79 ± 0.03 | 0.69 * |
Several studies have shown that C. Radix polysaccharides have bioactive properties. For example, C. Radix polysaccharides were shown to inhibit the growth of human gastric adenocarcinoma cells and hepatoma carcinoma cells [10], and stimulate concanavalin A or lipopolysaccharide induced lymphocyte proliferation [2]. Although there are few reports on the bioactivities of C. Radix volatiles, they are considered as important components [6]. Sulfur fumigation was shown to significantly alter the volatile oil and polysaccharide composition of C. Radix samples [6]. In our study, the contents of the main volatile oils and total polysaccharides were reduced by PGR treatment. This may be because the increase in yield under PGR treatment directed resources away from secondary metabolism. Since both growth and secondary metabolism consume nutrients and energy, an increase in yield can lead to a decrease in quality.
2.3. General Increase in Free Amino Acids Content by PGR Treatment
The contents of 24 common free amino acids in C. Radix were determined. The majority of free amino acids showed higher contents in the PGR-treated samples than in the control samples, especially asparagine, glutamic acid, α-aminoadipic acid, and ornithine (Table 2). The glycine content was significantly lower in the PGR-treated samples than in the control samples.
Table 2
Effect of PGR on free amino acids in C. Radix samples.
Free Amino Acid | Treatment (μg/g) | Control (μg/g) | T/CK |
---|---|---|---|
Glutamic acid | 3234.18 ± 811.83 | 465.59 ± 174.63 | 6.95 * |
Asparagine | 172.88 ± 44.88 | 34.36 ± 15.40 | 5.03 * |
α-Aminoadipic Acid | 3270.40 ± 898.05 | 659.44 ± 244.17 | 4.96 * |
Ornithine | 13.08 ± 1.59 | 6.41 ± 2.04 | 2.04 * |
Taurine | 121.03 ± 85.58 | 7.56 ± 5.38 | 16.01 |
Phosphatidylserine | 32.91 ± 16.27 | 10.05 ± 1.71 | 3.27 |
Carbamide | 17619.48 ± 7948.53 | 9652.14 ± 3208.69 | 1.83 |
Leucine | 22.65 ± 7.09 | 12.91 ± 4.08 | 1.75 |
Arginine | 5431.27 ± 1205.33 | 3164.94 ± 1227.21 | 1.72 |
Serine | 47.96 ± 11.59 | 32.21 ± 12.45 | 1.49 |
Citrulline | 214.58 ± 74.13 | 149.97 ± 60.34 | 1.43 |
β-Aminoisobutyric acid | 1.72 ± 0.42 | 1.28 ± 0.58 | 1.34 |
Valine | 12.34 ± 2.41 | 9.42 ± 3.65 | 1.31 |
Isoleucine | 61.95 ± 20.73 | 51.04 ± 16.87 | 1.21 |
Lysine | 50.74 ± 9.78 | 44.94 ± 18.04 | 1.13 |
β-Alanine | 4.93 ± 2.66 | 4.47 ± 2.49 | 1.10 |
Phenylalanine | 18.95 ± 4.69 | 17.45 ± 5.79 | 1.09 |
Alanine | 270.21 ± 102.56 | 291.28 ± 111.14 | 0.93 |
Glycine | 0.00 ± 0.00 | 3.20 ± 1.10 | 0.00 * |
Tyrosine | 8.98 ± 2.76 | 11.19 ± 4.72 | 0.80 |
Histidine | 2448.93 ± 786.52 | 2454.37 ± 360.11 | 1.00 |
γ-Aminobutyric acid | 27.76 ± 8.04 | 44.82 ± 10.31 | 0.62 |
Tryptophan | 21.32 ± 8.14 | 37.35 ± 17.81 | 0.57 |
Threonine | 9.96 ± 5.08 | 45.86 ± 53.54 | 0.22 |
Free amino acids play important roles in gene expression, protein synthesis, cell signaling, metabolism, physiology, and health [16]. Many of the amino acids in C. Radix may be related to its effects to promote metabolism and enrich the blood and saliva. Since the main functional components of the PGR we used are mainly quaternary ammonium compounds and nitrogen-containing heterocyclic compounds, it can inhibit the biosynthesis of gibberellins [17]. This reduces the division, elongation, and growth rates of meristem cells, leading to increased tuber growth and an increased yield. These nitrogen-containing compounds can be used as substrates for amino acid synthesis. This explains why the amino acids contents generally increased after treated with PGR.
2.4. Application of PGR Altered the Composition of Secondary Metabolites in C. Radix
A principal component analysis (PCA) was used to identify differences between the PGR treated and control samples (Figure 3A–D). We detected substantial differences in all conditions, except for the C18 column with ESI+. Then, we used a S-Plot analysis to select small-molecule metabolites showing significant differences in abundance between the PGR-treated and untreated groups (Figure 3E–G). The 15 metabolites showing major differences in abundance were listed in Table 3 and Table 4. Eleven of them were up-regulated by PGR treatment (Table 3) and four of them were down-regulated by PGR treatment (Table 4).

Effect of PGR on secondary metabolites in C. Radix samples. CK, untreated C. Radix sample; T, PGR-treated C. Radix sample. (A–D) Principal component analysis (PCA) of secondary metabolites. (E–G) S-plot analysis of secondary metabolites, red marked points were selected for further identification. (A, E) Chromatographic separation performed using ACQUITY UPLC BEH Amide column in ESI negative mode. (B, F) Chromatographic separation performed using ACQUITY UPLC BEH Amide column in ESI positive mode. (C, G) Chromatographic separation performed using ACQUITY UPLC HSS T3 C18 column in ESI negative mode. (D) Chromatographic separation performed using ACQUITY UPLC HSS T3 C18 column in ESI positive mode.
Table 3
Major secondary metabolites up-regulated by PGR.
No. | Identification and Tentative Identification | Molecular Formula | RT (min) | m/z | T/CK | Mode |
---|---|---|---|---|---|---|
1 | Arginine | C6H14N4O2 | 0.70 | 173.1045[M − H]− | 1.57 * | C18, ES− |
2 | Verbascose | C30H52O26 | 0.75 | 827.2670[M − H]− | 1.71 ** | C18, ES− |
3 | (4-Aminomethyl-1,2,3-triazol-1-acetylamino)-5-hydroxybenzoic acid | C12H13N5O4 | 0.82 | 290.0883 [M − H]− | 2.23 ** | C18, ES− |
4 | 4-Mannopyranosyl-5-O-phosphono-ribitol | C11H23O12P | 0.82 | 377.0859 [M − H]− | 1.62 * | C18, ES− |
5 | Acetylneuraminyl-galactose | C17H29NO14 | 0.82 | 470.1523 [M − H]− | 2.67 ** | C18, ES− |
6 | Deamino neuraminyl-galactosyl-acetyl glucosamine | C23H39NO19 | 0.82 | 632.2041 [M − H]− | 3.19 ** | C18, ES− |
7 | Glycoloyloxy-acetic acid | C4H6O5 | 0.87 | 133.0144 [M − H]− | 1.51 ** | C18, ES− |
8 | Bougainvillein-γ-I | C30H36N2O18 | 11.68 | 729.2257[M − NH4]− | 1.25 | Amide, ES− |
9 | Aurin | C19H14O3 | 20.27 | 307.1207[M+NH4]− | 1.72 ** | Amide, ES− |
10 | 9-Methylthio-2-nonanoic acid | C10H18O3S | 20.32 | 217.0906 [M − H]− | 1.38 ** | Amide, ES− |
11 | 5,6,7,8-Tetrahydromethanopterin | C30H45N6O16P | 7.20 | 794.3002[M+NH4] | 6.29 ** | Amide, ES+ |
Table 4
Major secondary metabolites down-regulated by PGR.
No. | Identification and Tentative Identification | Molecular Formula | RT (min) | m/z | T/CK | Mode |
---|---|---|---|---|---|---|
1 | 3-Hexadecanoylamino-2-hydroxy-4-methylpentyl-phosphonic acid | C22H46NO5P | 1.05 | 453.3469[M + NH4] | 0.02 ** | Amide, ES+ |
2 | 4,4′-Dioxo-carotene-3,3′-diyl didecanoate | C60H88O6 | 1.05 | 905.6666[M + H]+ |
![]() ![]() ![]() | ||
ORIGINAL ARTICLE | ||
|
Determination of ruscogenin in ophiopogonis radix by high-performance liquid chromatography-evaporative light scattering detector coupled with hierarchical clustering analysis
Chun-Hua Liu1, Ming Li1, Ya-Qian Feng1, Yuan-Jia Hu2, Bo-Yang Yu3, Jin Qi1
1 Department of Complex Prescription of TCM, Jiangsu Key Laboratory of TCM Evaluation and Translational Research, China Pharmaceutical University, Nanjing 211198, PR China
2 State Key Laboratory of Quality Research in Chinese Medicine, Institute of Chinese Medical Sciences, University of Macau, Macao 999078, PR China
3 Department of Complex Prescription of TCM, Jiangsu Key Laboratory of TCM Evaluation and Translational Research; State Key Laboratory of Natural Medicines, China Pharmaceutical University, Nanjing 211198, PR China
Date of Web Publication | 10-Feb-2016 |
Correspondence Address:
Bo-Yang Yu
Department of Complex Prescription of TCM, Jiangsu Key Laboratory of TCM Evaluation and Translational Research and State Key Laboratory of Natural Medicines, China Pharmaceutical University, Nanjing 211198
PR China
Jin Qi
Department of Complex Prescription of TCM, Jiangsu Key Laboratory of TCM Evaluation and Translational Research, China Pharmaceutical University, Nanjing 211198
PR China
Source of Support: None, Conflict of Interest: None
DOI: 10.4103/0973-1296.176008

![]() |
Background: Ophiopogonis Radix is a famous traditional Chinese medicine. It is necessary to establish a suitable quality control methods of Ophiopogonis Radix. Objective: To investigate the quality control methods of Ophiopogonis Radix by high-performance liquid chromatography (HPLC) coupled with evaporative light scattering detector (ELSD). Materials and Methods: A rapid and simple method, HPLC coupled with ELSD, was applied to determinate ruscogenin in 35 batches of Ophiopogenis Radix samples. Orthogonal tests and single factor explorations were used to optimize the extraction condition of ruscogenin. The content of ruscogenin in different origin was further analyzed by hierarchical clustering analysis (HCA). Results: The ruscogenin was successfully determined by HPLC-ELSD with a two-phase solvent system composed of methanol-water (88:12) at a flow rate 1.0 ml/min, column temperature maintained at 25°C, detector draft tube temperature at 42.2°C, nebulizer gas flow rate at 1.4 L/min, and the gain at 8. The result showed the good linearity of ruscogenin in the range of 40.20-804.00 μg/ml (R2 = 0.9996). Average of recovery was 101.3% (relative standard deviation = 1.59%). A significant difference of ruscogenin content was shown among 35 batches of Ophiopogenis Radix from different origin, varied from 0.0035% to 0.0240%. HCA based on the content of ruscogenin indicated that Ophiopogonis Radix in different origin was mainly divided into two clusters. Conclusion: This simple, rapid, low-cost, and reliable HPLC-ELSD method could be suitable for measurement of ruscogenin content rations and quality control of Ophiopogonis Radix.
Keywords: Hierarchical clustering analysis, high-performance liquid chromatography-evaporative light scattering detector, Ophiopogonis Radix, ruscogenin
How to cite this article: Liu CH, Li M, Feng YQ, Hu YJ, Yu BY, Qi J. Determination of ruscogenin in ophiopogonis radix by high-performance liquid chromatography-evaporative light scattering detector coupled with hierarchical clustering analysis. Phcog Mag 2016;12:13-20 |
How to cite this URL: Liu CH, Li M, Feng YQ, Hu YJ, Yu BY, Qi J. Determination of ruscogenin in ophiopogonis radix by high-performance liquid chromatography-evaporative light scattering detector coupled with hierarchical clustering analysis. Phcog Mag [serial online] 2016 [cited 2020 Sep 25];12:13-20. Available from: http://www.phcog.com/text.asp?2016/12/45/13/176008 |
Summary
- Ophiopogonis Radix is an important Traditional Chinese Medicine (TCM) to treat and prevent cardiovascular diseases and acute or chronic inflammation for thousands of years. Steroidal saponins were known as the dominant active components for their significant cardiovascular activity, and the most steroid sapogenin of them is ruscogenin. Therefore, ruscogenin was chosen as the marker component for evaluating the quality of Ophiopongonis Radix. This study focused on establishing a stable, low-cost, simple and practical method of HPLC-ELSD to determine the ruscogenin content, and 35 batches of samples of Ophiopogonis Radix were determined. Meanwhile, these results were analyzed by hierarchical clustering analysis and the methodology validation was based on USP34-NF-29 <1225>. Results showed that this analysis method was simple and stable, which would provide an important reference to establish the quality control methodology for other herb preparations and formulas containing Ophiopogonis Radix.
![]() | ![]() |
Ophiopogonis Radix, a popular traditional Chinese medicine (TCM), was used to treat and prevent cardiovascular diseases and acute or chronic inflammation for thousands of years, [1],[2] consisting of the dried tuberous root of Ophiopogonjaponicus (L.f.) Ker-Gawl (known as Maidong). [3]O.japonicus is an evergreen perennial widely distributed in South China, especially in Zhejiang Province (Zhe Maidong in China) and Sichuan Province (Chuan Maidong in China). [2],[4]
On the basis of previous phytochemical studies, steroidal saponins, homoisoflavonoids, and saccharides have been reported as the major components in O.japonicas. [5],[6],[7],[8],[9] In these components, steroidal saponins were known as the dominant active components for their significant cardiovascular activity, [1] and the most steroid sapogenin of them is ruscogenin [Figure 1]. [10] Ruscogenin was used to treat acute and chronic flammatory diseases [11],[12] and cardiovascular diseases such as arrhythmia, angina, and thrombosis. [13],[14],[15] To ensure their clinical efficacy, quality control of O.japonicus is of great importance. Given this, ruscogenin was chosen as the marker component for evaluating the quality of Ophiopogonis Radix.
Ruscogenin along with its glycoside saponins are difficult to be detected by UV detector due to the lack of UV chromophore. ELSD is a universal and nonspecific mass detector based on the detection of solute molecules by light scattering after nebulization and evaporation of the mobile phase. [16],[17] Therefore, high-performance liquid chromatography-evaporative light scattering detector (HPLC-ELSD), a practical and low-cost method, serves as a complementary tool for the detection of steroid sapogenin.
In the past years, it is a challenge for the quality control of Ophiopogonis Radix because of the lower content of individual steroidal saponin and homoisiflavonoid. Although they may be detected by mass spectrometry (MS), [6] there are no enough equipments in every laboratory because of immense expense. ELSD could overcome this problem. Several studies have been conducted on the determination of Ophiopogonin D or Ophiopogonin D' by ELSD, [18],[19] total saponins by UV-VIS spectrophotometer, [8],[20],[21] homoisoflavonoids by HPLC, [22] diosgenin, and ruscogenin by nonaqueous capillary electrophoresis, [23] and fingerprint analysis have been used in some investigations. [17],[24] However, until now few studies [25] focus on determination of ruscogenin in Ophiopogonis Radix by HPLC-ELSD.
In the present study, the total ruscogenin after hydrolysis was determined by HPLC-ELSD, suitable extraction conditions of ruscogenin were systematically optimized, the content in different origin was analyzed and the quality of Ophiopogonis Radix was evaluated combining hierarchical clustering analysis (HCA).
![]() | ![]() |
Plant materials
Thirty-five batches of Ophiopogenis Radix were collected from different provinces in China, including Jiangsu, Anhui, Guizhou, Yunnan, Zhejiang, Guangxi, Sichuan, Chongqing, Jiangxi, and Hunan. All samples were identified by Prof. Bo-Yang Yu, the voucher specimen (Number: 20111008) was deposited in the Herbarium of Department of Traditional Chinese Medicine of China Pharmaceutical University. Standard of ruscogenin was prepared in our laboratory and identified with purity no <98%.
Chemicals
HPLC-grade methanol was purchased from Yuwang Group (Shandong, China); ultrapure water was prepared by Milli-Qsystem (Milford, MA, USA); other chemicals and solvents were analytical grade. Sulfuric acid was bought from Nanjing Chemical Reagent Co., Ltd. (Jiangsu, China); sodium hydroxide was bought from Xilong Reagent Co., Ltd. (Guangdong, China); diethyl ether was bought from Nanjing Chemical Reagent Co., Ltd. (Jiangsu, China).
Instruments
Agilent 1260 HPLC System (Agilent Technologies, Palo Alto, CA, USA) comprises a quaternary solvent delivery system, an on-line degasser, an auto-sampler, a column temperature controller, and an analytical workstation (Agilent LC B.04.03 ChemStation) coupled with detector Alltech 3300 ELSD (Alltech, USA).
Chromatographic conditions
The analytical column was a Merck RP-C 18 (4.6 mm × 250 mm, 5 μm) with C 18 guard column. The mobile system consisted of methanol-water (88:12) at a flow rate of 1.0 ml/min. Column temperature was maintained at 25°C. The draft tube temperature was at 42.2°C, the nebulizer gas flow rate was at 1.4 L/min, the gain was at 8. The solvent was filtered through a 0.22 μm filter and degassed. The sample injection volume was 10 μl. The total acquisition time was 25 min.
Reference standard solution preparation
Standard stock solutions with known concentrations were obtained by dissolving accurately weighted ruscogenin in methanol.
Optimization of extraction condition
As, the extraction of ruscogenin was affected by many factors, so orthogonal designs were taken to optimize the extraction condition, including optimization of acid hydrolysis conditions and extraction of ruscogenin.
Optimization of acid hydrolysis conditions
According to related literatures and previous preliminary experiments, [25] orthogonal design 1 was applied to select the acid hydrolysis conditions of ruscogenin in Ophiopogonis Radix. 2.0 g Ophiopogonis Radix was transferred to a suitable flask, and 25 ml sulfuric acid with different concentration was respectively added to hydrolysis for different time in water bath with different temperature according to factors [Table 1]. Then the extracting solution was adjusted to neutral with 16% NaOH and filtered. The residue was collected and dried at 60°C and then soxhlet extracted with 70 ml petroleum ether for 6 h. Petroleum ether layer was collected and evaporated to dryness. Then the residue was transferred to a 2 ml volumetric flask and diluted to 2 ml with methanol. The obtained solution was passed through a membrane filter (0.45 μm), and the first part of the filtrate was discarded, then the successive filtrate was collected as the sample solution. Ten microliters sample solution and standard solution were injected into the chromatography, in which the chromatograms were recorded, and the content of rescogenin was calculated in Ophiopogonis Radix coupled with visual analysis and variance analysis. The results of the orthogonal test and extreme difference analysis were presented in [Table 2]. All the analysis of variance were performed by the statistical software SPSS version 19.0 (SPSS Inc., Chicago, Illinois, USA) and the result was listed in [Table 3].
Optimization of extraction of ruscogenin
Orthogonal design 2 was applied to select the best extraction condition of ruscogenin. During this procedure, the steps before being extracted with petroleum ether were similar to "Optimization of acid hydrolysis conditions," and just the acid hydrolysis was performed using certain factors with 25 ml 3% H 2 SO 4 and being refluxed in boiling water bath for 6 h. After being adjusted to neutral with 16% NaOH and filtered, residue was collected and dried at 60°C and then extracted with 70 ml different solvents, different methods, and different time as factors [Table 4]. The rest steps were same as the corresponding process of "Optimization of acid hydrolysis conditions." The results of orthogonal test 2 and extreme difference analysis were presented in [Table 5]. The analysis of variance [Table 6] was performed by statistical software SPSS version 19.0 (SPSS Inc.).
Single factor experiment
To select better extraction method, the different hydrolysis time was investigated based on orthogonal design 1, compared in these experiments (4 h, 6 h) as shown in [Table 7], and the different extraction solvents (cyclohexane, ether) were analyzed on the basis of orthogonal experiment 2 [Table 8].
Sample solutions preparation
Sample solutions preparation were based on above optimized conditions as following: About 2.0 g Ophiopogons Radix, powdered to pass through a 24 mesh sieve and accurately weighed, was transferred to a 100 ml glass-stoppered conical flask. Twenty-five milliliters 3% H 2 SO 4 was accurately added to reflux in boiling water bath for 6 h. Extracting solution was adjusted to neutral with 16% NaOH and then filtered. The collected residue, dried at 60°C, was soxhlet extracted with 70 ml ether for 6 h. Ether layer was collected, and evaporated to dryness and the residual were transferred to a 2 ml volumetric flask and diluted to 2 ml with methanol, which passed through a membrane filter (0.45 μm porosity), discarding the first part of the filtrate and collecting the successive filtrate as the sample solution. Ten microliters sample solution and standard solution were injected into the chromatography as "Chromatographic conditions," in which the chromatograms were recorded, and the content of ruscogenin of Ophiopogonis Radix was calculated. Thirty-five batches of Ophiopogonis Radix samples were extracted with this same method.
Statistical analysis
Statistics was analyzed with SPSS version 19.0 statistical software (SPSS, USA).
![]() | ![]() |
Optimization of extraction condition
The results [Table 2] showed that hydrolysis temperature has a notable influence on the acid hydrolysis of saponins from O. japonicus. Besides, visual analysis [Table 2] stated that the influence to the mean extraction yields of the ruscogenin decreases in the order: B > A ≥ C according to the R values, and the best combination of test factors was A2B3C1. However, based on analysis of variance [Table 3], A, B, and C have no significant difference. Therefore, to ensure the repeatability and stability of the method, further experiments as "Investigation of hydrolysis time" were applied to optimize the hydrolysis time.
Optimization of extraction of ruscogenin
Extraction time have a notable influence on the extraction of ruscogenin [Table 5]. Besides, visual analysis [Table 5] stated that the influence to the mean extraction yields of the ruscogenin decreases in the order: C > B > A according to the R values, and the best combination of test factors was A2B1C3 or A3B1C3. However, based on analysis of variance [Table 6], A, B and C have no significant difference. Therefore, to select the best extraction method and ensure the stability, further experiments were performed to compare A2B1C3 with A3B1C3 as "Investigation of extraction solvents."
Single factor experiment
Investigation of hydrolysis time
The results [Table 7] showed that 6 h was appropriate for hydrolysis because its relative standard deviation (RSD) was not more than 2.0%. So, the optimal conditions were determined: 25 ml, 3% sulfuric acid concentration, refluxed in the boiling water bath for 6 h.
Investigation of extraction solvents
Solution ether was appropriate [Table 8] because of its RSD < 2.0% as well as relatively high content of ruscogenin, so ether was chosen for further study.
Determination of ruscogenin in Ophiopogonis Radix
Method validation of ruscogenin: Precision, linearity, accuracy, specificity, stability, detection limit, quantitation limit, and ruggedness.
The method was validated through investigation on the precision, linearity, accuracy, specificity, stability, and ruggedness.
Precision (repeatability and intermediate precision)
Repeatability was assessed with 9 sample solutions of low, medium, and high levels (1.0, 2.0 and 3.0 g respectively), each with three triplicates. The content of ruscogenin was calculated with the external reference method [Table 9]. The RSDs of inner-day variation of three levels were in the range from 0.84% to 4.40% for the content of ruscogenin, and average RSD was 3.53%.
Intermediate precision was performed through investigation of 3 different days, 3 different analysts and 3 different columns, each with 3 triplicates [Table 10], [Table 11] and [Table 12]. These results showed good precision.
Linearity and range
Good linearity was shown with correlation 0.9996 [Table 13].
Accuracy
Accuracy was calculated as the percentage of recovery by the assay of the known added amount of ruscogenin in the sample.
Three different concentrations (low, medium, and high) of reference standard were spiked to 2.0 g of Ophiopogonis Radix, all in triplicates. Average recovery was 101.32% [Table 14].
Specificity
The integration peak in the chromatogram of the sample solution was corresponding in time to the peak in the chromatogram of standard solution. No such peak of that retention time appeared in the chromatogram of blank solvent [Figure 2].
![]() | Figure 2: Specificity, (a) Standard solution; (b) Sample solution; (c) Blank solution Click here to view |
Stability
The stability of sample solution was investigated by comparing the peak areas and retention time of ruscogenin in the chromatograph of the same sample solution, after stored at room temperature for different time (0, 2, 4, 6, 8, 12, 24 h). Stability was evaluated by calculating the RSD of peak area and retention time. The RSD were 0.61% and 0.46%, respectively [Table 15]. The analyses were found to be stable within 24 h in the sample solution.
Detection limit
According to USP34 NF 29 chapter <1225>, detection limit was 0.0804 μg/ml and when the concentration was 0.0804 μg/ml, signal to noise ratios were about 3:1.
Quantitation limit
According to USP34 NF 29 chapter <1225>, when the concentration was 0.1608 μg/ml, signal to noise ratios were about 10:1. Quantitation limit was 0.1608 μg/ml.
Ruggedness
The ruggedness of the established method was evaluated by examining its stability with small variations of procedural parameters, including pH of mobile phase, ratio of components in mobile phase, ELSD detector, different columns, flow rate, injection volume, and column temperature.
Results of ruggedness indicated that the drift tube temperature of ELSD should be not lower than 42.2°C; the pH of mobile phase, ratio of components in mobile phase, flow rate, injection volume, and column temperature can be slightly adjusted for system suitability.
Content of ruscogenin in 35 batches of Ophiopogonis Radix
Sample solutions of 35 batches of Ophiopogonis Radix were determined using the validated chromatographic method for ruscogenin. Its content varied from 0.0035% to 0.0240% [Table 16], [Figure 3] and [Figure 4].
![]() | Figure 4: Content of ruscogenin in Ophiopogonis Radix in different origin Click here to view |
Hierarchical clustering analysis
In order to assess the variation of Ophiopogonis Radix in different origin, HCA was performed based on the content of rescogenin from HPLC-ELSD profiles. Between groups linkage method was performed, and Squared Euclidean distance was selected as a measurement. In HCA dendrogram, the shorter distance between two samples indicated their higher similarity and the samples clustered into the same group were the most similar ones. [26] The results [Figure 5] demonstrated that the 35 batches of samples were obviously divided into two main clusters according to their contents. Sample 1-19 were belonging to cluster-I, which were mainly commercial Ophiopogonis Radix (Chuan Maidong) although collected from different province, whereas sample 20-35 were belonging to cluster-II, which were mainly collected from Zhejiang Province and partly from cultivation base in Sichuan Province.
![]() | Figure 5: Dendrograms of hierarchical cluster analysis for the 35 tested samples of Ophiopogonis Radix Click here to view |
![]() | ![]() |
Optimization of high-performance liquid chromatography-evaporative light scattering detector condition
HPLC, a simple, stable, and durable analysis techniques, is widely applied to assess the types and content of chemical compositions in TCMs. HPLC can be equipped with multiple detectors, such as a UV detector, ELSD, fluorescence detector and MS, of which the UV detector is most frequently used. [27] Given that ruscogenin lack of UV chromophore, HPLC coupled with ELSD was applied to our research. In order to achieve rapid and stable analysis of ruscogenin in Ophiopogonis Radix, ELSD different nebulizer-gas flow rate (1.3, 1.4, 1.5, 1.6 L/min), and drift tube temperature (39.2°C, 40.6°C, 42.2°C, and 45°C) were compared according to the value of signal-to-noise ratio. These results indicated that the value of signal-to-noise ratio was better when the nebulizer-gas flow rate was at 1.4 L/min and drift tube temperature was at 42.2°C. Therefore, they were chosen for the analysis.
To obtain the chromatograms with the good separation, different chromatographic condition, including mobile phase system (methanol- and acetonitrile-water), pH of mobile phase (water, 0.02% aqueous solution of formic acid, 0.10% aqueous solution of formic acid, and 0.3% aqueous solution of triethylamine), methanol ratio (85%, 88%, and 90%), flow rate (1.0, 1.1, and 1.2 ml/min), column temperature (15°C, 25°C, and 30°C), and injection volume (5, 10, 15 and 20 μl) were compared and analyzed. As a result, based on system suitability parameters (tailing factor, resolution, and number of theoretical plates), methanol-water (88-12), flow rate 1.0 ml/min, column temperature 25°C, and injection volume 10 μl were finally chosen.
Optimization of sample preparation
In order to obtain better extraction condition, variables involved in the procedure such as hydrolysis and extraction method (extraction firstly, then hydrolysis) and different acid (hydrochloric acid, sulfuric acid) were optimized in preliminary experiment. About 2.0 g Ophiopogonis Radix, powdered to pass through a 24 mesh sieve, accurately weighed, was soxhlet extracted with 70 ml methanol for 4 h once, then solution was collected, evaporated to dryness, and residue was hydrolyzed with 25 ml 3% H 2 SO 4 in water bath for 4 h, and 16% NaOH was added to adjust neutral. Then the hydrolysate was extracted with 50 ml petroleum ether for 3 times, and petroleum ether layer was collected and evaporated to dryness. The rest steps were similar with corresponding programs in "Sample solutions preparation." This result suggested that more impurity was included in a sample solution with this method, compared with the procedure of hydrolsis firstly, then extraction as "Optimization of extraction condition" of the methods section. Moreover, the preliminary experiment also investigated the hydrolysis of different acid (2.22% hydrochloric acid, 3% sulfuric acid), the other steps was same as corresponding "Optimization of extraction condition" of methods section. The result indicated that content of rescogenin with 3% sulfuric acid was more stable (the RSD was <2%, while more than 10% with hydrochloric acid, n = 3). Thus, sulfuric acid was chosen for further experiments. Based on these preliminary experiments, orthogonal tests, and single factor explorations were designed in a present experiment to select the best extraction condition.
Validation of methodology
According to USP34-NF-29 chapter <1225>, precision (repeatability and intermediate precision), linearity, accuracy, specificity, stability, detection limit, quantitation limit, and ruggedness were investigated. Average RSD of repeatability was 3.53%; average RSD of intermediate precision-different days, different analysts and different column were 3.71%, 3.75%, and 1.29%, respectively. The calibration curves exhibited good linearity (R2 = 0.9996) in the range of 40.20-804.00 g/ml. Average of recovery was 101.3% (RSD = 1.59%, n = 9). Stability RSD of the area and retention time was 0.61% and 0.46%. The detection limit was 0.0804 μg/ml and quantitation limit was 0.1608 μg/ml. Results of ruggedness indicated that the drift tube temperature of ELSD detector (Alltech ELSD 3300) should be not lower than 42.2°C. The pH of mobile phase, the ratio of components in the mobile phase, flow rate, injection volume, and column temperature can be slightly adjusted for system suitability. These indicated that the analysis method was simple, feasible, and stable.
Hierarchical clustering analysis
Based on the content of ruscogenin in Ophiopogonis Radix, HCA was performed to analyze results of 35 batches of samples (Ophiopogonis Radix). These results illustrated that samples could be appropriately divided into two main clusters related to their contents and the difference of content in samples was significant in a different origin. It is well known that types and quantities of chemical components in medicinal plants are probably considerably affected on collection at different times and from different localities. [26],[28] This reason is related to different environments, soil texture condition, growing time, harvesting time, and cultivated techniques. Therefore, in order to control the quality of Ophiopogonis Radix, it is necessary to establish a stable and simple analysis method. In the present study, a practical and the low-cost technique could evaluate the quality through systematically optimization of extraction condition, chromatographic condition, and method validation.
![]() | ![]() |
In the present work, an impersonal, valid, low-cost and the stable analysis method was developed and applied to the quality control of the Ophiopogonis Radix through systematically optimization of extraction condition, chromatographic condition and method validation. Sample solutions of 35 batches of Ophiopogonis Radix were determined using the validated chromatographic method for ruscogenin. The content of ruscogenin varied from 0.0035% to 0.0240%. Our results also showed that HPLC-ELSD enjoyed the practical advantages of simple analysis procedure and reduced quantity of cost consumption for the quality control of Ophiopogonis Radix. Therefore, the method developed in this study would provide an important reference to establish the quality control method for other herb preparations and formulas containing Ophiopogonis Radix.
Acknowledgements
The authors would like to thank the United States Pharmacopeia Convention and National Natural Science Foundation of China (No. 81274004 and No. 81473317) for technical and financial support, respectively.
Financial support and sponsorship
Nil.
Conflicts of interest
There are no conflicts of interest.
![]() | ![]() |
1. | Zhou YF, Qi J, Zhu DN, Yu BY. Homoisoflavonoids from Ophiopogon japonicus and its oxygen free radicals (OFRs) scavenging effects. Chin J Nat Med 2008;6:201-4. ![]() |
2. | Li N, Zhang JY, Zeng KW, Zhang L, Che YY, Tu PF. Anti-inflammatory homoisoflavonoids from the tuberous roots of Ophiopogon japonicus. Fitoterapia 2012;83:1042-5. ![]() |
3. | China SAoTCMotPsRo. Herbal of China. Shanghai: Shanghai Science and Technology Publishers; 1999. p. 122. ![]() |
4. | Qi J, Xu D, Zhou YF, Qin MJ, Yu BY. New features on the fragmentation patterns of homoisoflavonoids in Ophiopogon japonicus by high-performance liquid chromatography/diode-array detection/electrospray ionization with multi-stage tandem mass spectrometry. Rapid Commun Mass Spectrom 2010;24:2193-206. ![]() |
5. | Li N, Zhang L, Zeng KW, Zhou Y, Zhang JY, Che YY, et al. Cytotoxic steroidal saponins from Ophiopogon japonicus. Steroids 2013;78:1-7. ![]() |
6. | Xie T, Liang Y, Hao H, A J, Xie L, Gong P, et al. Rapid identification of ophiopogonins and ophiopogonones in Ophiopogon japonicus extract with a practical technique of mass defect filtering based on high resolution mass spectrometry. J Chromatogr A 2012;1227:234-44. ![]() |
7. | Zhang T, Kang LP, Yu HS, Liu YX, Zhao Y, Xiong CQ, et al. Steroidal saponins from the tuber of Ophiopogon japonicus. Steroids 2012;77:1298-305. ![]() |
8. | Zhou YH, Wu XR, Xu DS, Feng Y, Li JJ. Purification of total saponins of Ophiopogons Radix by macroporous resin. Chin Tradit Herb Drugs 2003;34:1089-91. ![]() |
9. | Wagner H, Bauer R, Melchart D, Xiao PG, Staudinger A. Radix Ophiopogonis - Maidong. In: Wagner H, Bauer R, Melchart D, Xiao PG, Staudinger A, editors. Chromatographic Fingerprint Analysis of Herbal Medicines. Springer Wien NewYork: Springer Vienna; 2011. p. 819-30. ![]() |
10. | Liu N, Wen X, Liu J, Liang M, Zeng H, Lin Y, et al. Determination of ruscogenin in crude Chinese medicines and biological samples by immunoassay. Anal Bioanal Chem 2006;386:1727-33. ![]() |
11. | Liu J, Chen T, Yu B, Xu Q. Ruscogenin glycoside (Lm-3) isolated from Liriope muscari inhibits lymphocyte adhesion to extracellular matrix. J Pharm Pharmacol 2002;54:959-65. ![]() |
12. | Wu F, Cao J, Jiang J, Yu B, Xu Q. Ruscogenin glycoside (Lm-3) isolated from Liriope muscari improves liver injury by dysfunctioning liver-infiltrating lymphocytes. J Pharm Pharmacol 2001;53:681-8. ![]() |
13. | Bi LQ, Zhu R, Kong H, Wu SL, Li N, Zuo XR, et al. Ruscogenin attenuates monocrotaline-induced pulmonary hypertension in rats. Int Immunopharmacol 2013;16:7-16. ![]() |
14. | Xiao PG. Modern Chinese Materia. Vol. 1. Beijing: Chinese Industry Press; 2002. p. 71-81. ![]() |
15. | Sun Q, Chen L, Gao M, Jiang W, Shao F, Li J, et al. Ruscogenin inhibits lipopolysaccharide-induced acute lung injury in mice: Involvement of tissue factor, inducible NO synthase and nuclear factor (NF)-kappaB. Int Immunopharmacol 2012;12:88-93. ![]() |
16. | Li FJ, Zhang HY, Li Y, Yu YJ, Chen YL, Xie MF, etal. Simultaneous identification and quantification of dextran 20 and sucrose in lyophilized thrombin powder by size exclusion chromatography with ELSD. Chromatographia 2012;75:187-91. ![]() |
17. | Li N, Che YY, Zhang L, Zhang JY, Zhou Y, Jiang Y, et al. Fingerprint analysis of Ophiopogonis Radix by HPLC-UV-ELSD coupled with chemometrics methods. J Chin Pharm Sci 2013;22:55-63. ![]() |
18. | Jia C, Ye ZL, Jiang XJ, Zhou DZ, Li DK. Quantitative determination of Ophiopogonin D and Ophiopogonin D' from Ophiopongon japonicus by ELSD-HPLC. Res Pract Chin Med 2012;26:79-81. ![]() |
19. | Yu JP, Ma YG, Shao JF, Zhu M. Quantitative determination of Ophiopongon D in Zhe Ophiopongon japonicus (Thunb.) Ker-Gawl. and Chuan Ophiopongon japonicus (Thunb.) Ker-Gawl. by HPLC-ELSD. Tradit Chin Drug Res Clin Pharmacol 2002;13:253-5. ![]() |
20. | P.R.China TSPCo. Pharmacopoeia of the People's Republic of China. 2010 th ed., Vol. 1. Beijing: People's Medical Publishing House; 2010. p. 144. ![]() |
21. | Tang XQ, Cao YX, Yu BY. Study on the quality control of Ophiopogons Radix. China J Chin Mater Med 1999;24:390-3. ![]() |
22. | Zeng P, Zhou H, Zheng Y, Xu X, Fu S. Simultaneous determination of three homoisoflavonoids in Ophiopogon japonicus by HPLC. Zhongguo Zhong Yao Za Zhi 2012;37:71-4. ![]() |
23. | Huang BM, Yao CW, Bian QQ, Wang ZG, Mo JY. Determination of diosgenin and ruscogenin in Radix Ophiopogonis by nonaqueous capillary electrophoresis. Yao Xue Xue Bao 2011;46:443-6. ![]() |
24. | Liu L, Lu Y, Shao Q, Cheng YY, Qu HB. Binary chromatographic fingerprinting for quality evaluation of Radix Ophiopogonis by high-performance liquid chromatography coupled with ultraviolet and evaporative light-scattering detectors. J Sep Sci 2007;30:2628-37. ![]() |
25. | Tang XQ, Yu BY, Xu DR, Tang Y, Xu LS. Determination of sapogenins in Radix Ophiopogonis by HPLC-ELSD. J China Pharm Univ 2001;32:270-2. ![]() |
26. | Liang X, Ma M, Su W. Fingerprint analysis of Hibiscus mutabilis L. leaves based on ultra performance liquid chromatography with photodiode array detector combined with similarity analysis and hierarchical clustering analysis methods. Pharmacogn Mag 2013;9:238-43. ![]() |
27. | Liang XM, Jin Y, Wang YP, Jin GW, Fu Q, Xiao YS. Qualitative and quantitative analysis in quality control of traditional Chinese medicines. J Chromatogr A 2009;1216:2033-44. ![]() |
28. | Kong WJ, Zhao YL, Xiao XH, Jin C, Li ZL. Quantitative and chemical fingerprint analysis for quality control of rhizoma Coptidischinensis based on UPLC-PAD combined with chemometrics methods. Phytomedicine 2009;16:950-9. ![]() |
![]() | ![]() |
Figures |
[Figure 1], [Figure 2], [Figure 3], [Figure 4], [Figure 5]
Tables |
[Table 1], [Table 2], [Table 3], [Table 4], [Table 5], [Table 6], [Table 7], [Table 8], [Table 9], [Table 10], [Table 11], [Table 12], [Table 13], [Table 14], [Table 15], [Table 16]
|
What’s New in the Radix 1.31 serial key or number?
Screen Shot
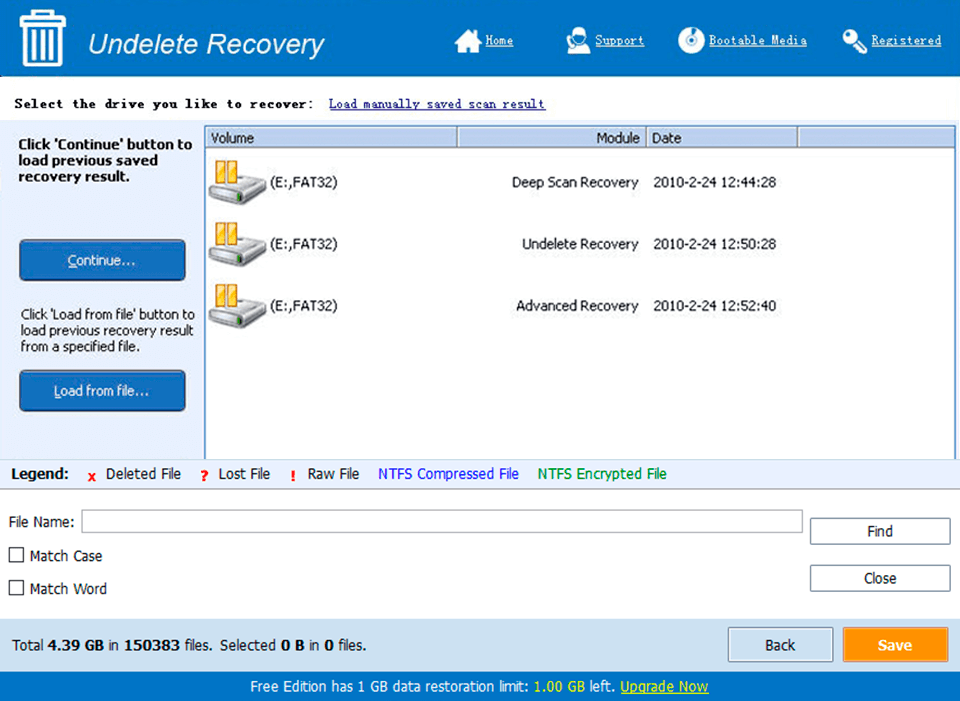
System Requirements for Radix 1.31 serial key or number
- First, download the Radix 1.31 serial key or number
-
You can download its setup from given links: