
Lab DJ 1.2.5 serial key or number
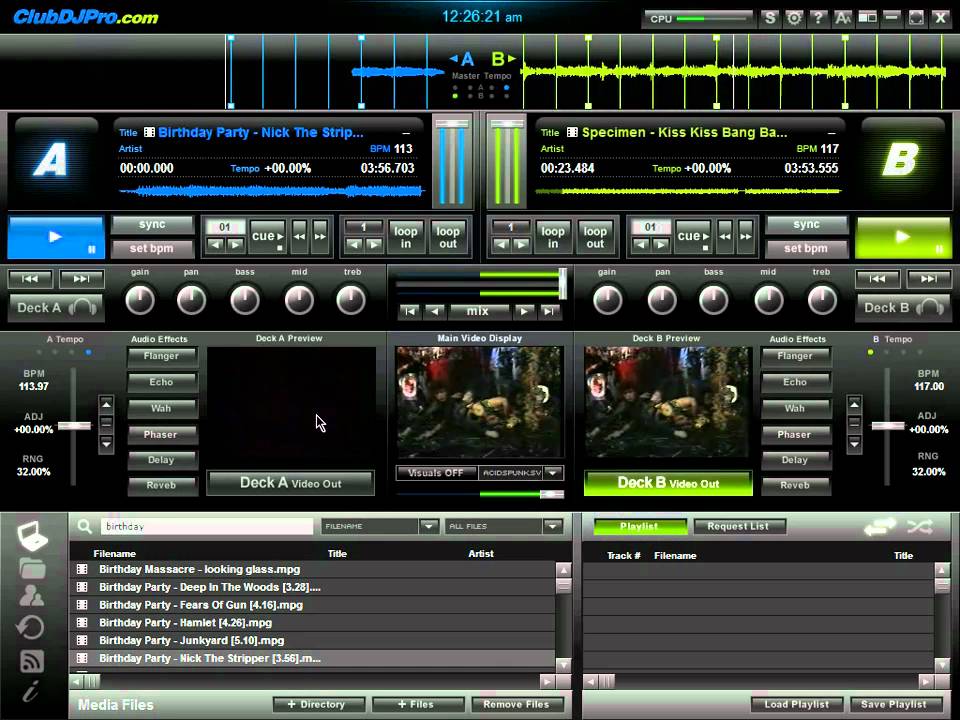
Lab DJ 1.2.5 serial key or number
Department of Health
A print friendly PDF version is available from this Communicable Diseases Intelligence issue's table of contents.
Background
This surveillance plan has been formulated to help prepare for national measles elimination. It updates and expands upon the surveillance methodology previously outlined in Measles: Guidelines for the control of outbreaks in Australia which was developed by the National Health and Medical Research Council (NHMRC).1 The NHMRC document also contains recommendations about individual case management and outbreak control, which will require revision once the Measles Control Campaign (MCC) has commenced. However, enhanced measles surveillance is needed as soon as possible, as the first phase of the MCC will take place between July and October, 1998.* Therefore, all jurisdictions should comply as closely as possible with these guidelines from 1 July 1998.These guidelines have been developed in collaboration with the Measles Elimination Advisory Committee and The Communicable Diseases Network of Australia and New Zealand. They are intended as best practice guidelines for all those who are likely to contribute towards measles surveillance and elimination in Australia, including: general practitioners, paediatricians and physicians, pathologists, diagnostic and public health laboratories, and disease control officers in State and Territory health departments.
As best practice guidelines, they assume resources that may not yet be available, but are needed for successful measles elimination. In particular, laboratory diagnostic methods and case investigation formats must be standardised, and an agreement made by all States and Territories that they collect the same minimum data set. Measles elimination requires coordinated efforts, perhaps more than any previous health initiative in Australia, and comprehensive surveillance is a critical element for success.
Footnote
* The first phase of the Measles Control Campaign was completed in the second half of 1998, after this article was accepted for publication. The results of the primary schools immunisation campaign have been reported in CDI. See for example Commun Dis Intell 1998;22:270. Prior to the campaign, NHMRC endorsed the change in timing of the second dose MMR which is now due prior to school entry at age 4 to 5 years.
Top of page
Introduction
In Australia, and worldwide, measles remains the leading cause of vaccine preventable deaths.2-4 Even with near universal single dose childhood vaccination it seems, with currently available vaccines, measles outbreaks can still occur.5 However, in the 1990s, major advances have been made in measles control, particularly in the Americas. Indigenous measles transmission has been interrupted in several Latin American countries, the English speaking Carribean, and the United States.6,7 In Latin America and the United Kingdom, measles control has been achieved through mass vaccination programs, administered regardless of vaccination history, to preschool and school-age children. In Finland and the United States, similar achievements have been attained by maintaining high coverage for a prolonged period with a two dose measles vaccination schedule.6 Substantial progress has also been achieved in the Western Pacific Region other than Australia.8 Mass campaigns are able to interrupt endemic transmission quite quickly. However, to prevent the reappearance or reintroduction of measles, very high routine vaccination coverage or smaller follow-up campaigns are needed.In July 1996, a joint meeting of the World Health Organization (WHO), the Centers for Disease Control and Prevention (CDC), and the Pan American Health Organisation was convened to consider the feasibility of global measles eradication.6 This group recommended the goal of global measles eradication, with a target date of 2005-2010. In July 1997, Australia's National Centre for Disease Control established a Measles Elimination Advisory Committee (MEAC). The MEAC provisionally recommended a national, predominantly school-based measles vaccination campaign to commence in the first quarter of 1999. It also recommended that after this campaign: the second dose of measles-mumps-rubella vaccine should be given prior to school entry, and the adolescent MMR program should cease in 1999. In April 1998, the Australian Technical Advisory Group on Immunisation, after considering implications for rubella immunisation, endorsed this change in the Standard Vaccination Schedule. To support these measles control initiatives, substantial enhancements of measles surveillance are required.
Measles elimination objectives
The principal objectives of the Australian measles elimination initiative are:- To cease measles related morbidity and mortality, by interrupting indigenous transmission of measles; and
- To prevent reintroduction of measles until global eradication is achieved, by maintaining uniformly low levels of population susceptibility.
Measles control targets
In order to achieve the elimination objectives outlined above, very high vaccination coverage and low susceptibility levels are needed, especially in closed settings such as schools where contact rates are high. Uniformity of coverage is also important, because pockets of susceptible persons are capable of perpetuating endemic transmission. The following vaccination coverage targets have been set, and should be pursued in all socioeconomic and ethnic groups, and in all regions.By 2000:
- 95 per cent coverage of school children with an additional dose of vaccine in a school based campaign;
- 80 per cent coverage of children with two doses of measles-containing vaccine by school entry.**
- 95 per cent coverage of children with one dose of measles containing vaccine by their second birthday (10% susceptibility);
- 95 per cent coverage of children with at least one dose, and 90 per cent with two doses of measles containing vaccine by school entry (5% susceptibility).
** This was the target prior to the campaign, although 90 per cent may now be more appropriate
Top of page
Measles Surveillance Tasks
Surveillance is an essential component of enhanced measles control initiatives. Very high quality active and passive surveillance is now necessary to determine whether measles elimination objectives and coverage targets are being achieved. In this strategy, measles surveillance tasks are described under the following headings:- Case definitions, diagnosis, and investigation.
- Enhancing surveillance.
- Outbreak investigation.
- Monitoring measles vaccination coverage and population susceptibility.
- Monitoring vaccine safety and effectiveness.
1. Case definitions, diagnosis, and investigation
For a measles elimination initiative, disease surveillance must fulfil several functions. In addition to measuring case rates and characterising populations at high risk for infection, we need to be able to:- Detect cases and the source of infection rapidly so that timely control measures can be implemented;
- Detect interruption or resurgence of indigenous measles transmission;
- Detect importation of measles;
- Monitor serious complications of measles infection (death, encephalitis, seizures, and pneumonia).
1.1. Measles case definitions
1.1.1. Suspected infection
A sensitive clinical definition is needed for the early detection of outbreaks and imported infection, and for timely interventions.A suspected case is an illness with all of the following featres: morbilliform rash, cough, and fever present at the time of rash onset.9
(The Pan American Health Organisation accepts any illness diagnosed as measles by a clinician as a suspected case. This more sensitive definition may need to be adopted as we approach elimination.6)
1.1.2. Laboratory confirmed infection
As measles becomes well controlled, the positive predictive value of clinical diagnosis becomes poor, especially for young children and sporadic disease, and laboratory based surveillance becomes increasingly important.10 Laboratory confirmation should be sought on all sporadic clinical notifications, and at least two cases during an outbreak. However, case investigation should not be delayed pending laboratory results (see section 1.3).Criteria for laboratory confirmation:
- A positive test for measles-specific IgM; or
- Isolation of wild measles virus from a clinical specimen; or
- A diagnostic rise in measles antibody titres in paired sera.
1.1.3. Rejected measles infection
A rejected case is an illness which is:- Initially categorised as suspected measles; and
- Subsequently found to have negative measles serology, and/or diagnosed as having an alternative cause based on laboratory evidence.
1.1.4. Epidemiological linkage
This category can provide additional evidence for measles infection in instances where laboratory confirmation is unavailable, or is equivocal (e.g. serodiagnosis following immunisation).
A measles case is epidemiologically linked if:
- There was exposure to a laboratory confirmed case during their infectious period (4 days before to 4 days after rash onset); and
- This exposure occurred within the expected incubation period of the case under investigation: 7-18 days (mean 14 days) before rash onset.11
1.1.5. Imported infection
Importation of infection poses an ongoing risk during the elimination phase of measles control. An increasingly large proportion of measles notifications in Britain and in the USA are attributable to imported infection.10,12International importation
A confirmed case whose rash onset is within 18 days of arrival in Australia.The last country visited prior to arrival in Australia should be recorded on the case investigation form (Appendix A).
All other cases are considered indigenous. All indigenous cases are further categorised as either epidemiologically linked to an internationally imported case (see above definition of linkage); or not linked epidemiologically to an internationally imported case.13
Top of page
Interstate importation
A confirmed case whose rash onset is within 18 days of entering the State or Territory. All other cases are considered local to the State or Territory.These definitions are intended to maximise detection of importation, and therefore will incorrectly label some locally acquired infections as imported.***
***A more specific definition for interstate importation is recommended by the Centers for Disease Control: A confirmed case who was outside the State /Territory for the entire incubation period (7-18 days before rash onset).14
1.2. Laboratory diagnosis
1.2.1. Serological diagnosis
Serum anti-measles IgM antibody testing is recommended for diagnosis of acute measles infection. The indirect enzyme immunoassay (EIA) is recommended for routine laboratory diagnosis, because it is relatively quick and convenient to perform. The test characteristics of commercially available indirect IgM EIAs are variable. The sensitivity and specificity of one such assay were estimated to be 86 per cent and 81 per cent respectively.15 Until further data are available, any of the commercially available kits for measles IgM are considered satisfactory for routine diagnosis.Timing of specimen collection
Detailed data regarding the optimum timing of specimens for IgM serology has been obtained using a measles capture IgM assay developed by the Centers for Disease Control and Prevention (CDC). This assay was frequently positive at the onset of rash illness, about 80 per cent sensitive within 72 hours of onset, 100 per cent between 4-14 days, falling to 94 per cent at 4 weeks and 64 per cent at 6 weeks.16 Therefore, a negative EIA test for IgM on serum sampled more than 72 hours after rash onset is very reliable, especially when measles is rare. However, when initial anti-measles IgM antibody is negative, but serum was sampled within the first 72 hours of rash onset, repeat serum sampling for IgM and IgG estimation is recommended after 14 days (range 10-30 days).Blood collection requirements
Laboratories generally require a minimum of 1mL clotted blood for serology. Blood can be tested from a finger-prick or heel-prick, but venipuncture is less traumatic in the hands of an experienced person. The testing laboratory should be consulted if doubts exist regarding the minimum volume of blood required. It is also possible to test blood which has been collected onto filter paper and air-dried, but this method is not routinely available in Australia.1.2.2. Confirmatory testing and quality assurance
As the incidence of true measles declines, so too will the positive predictive value of measles serodiagnosis; while the reliability of a negative test improves. Confirmatory testing of IgM positive cases will be needed to achieve acceptable diagnostic accuracy. In Australia, during inter-epidemic periods, all measles IgM positive and equivocal sera should be forwarded to a reference laboratory for confirmatory testing. During measles outbreaks, when positive tests are more likely to be reliable, a random sample only of IgM positive sera should be forwarded.In Australia, the recommended reference laboratory confirmatory test for acute measles infection is the IgM capture EIA assay. This assay has been evaluated by the Centers for Disease Control and Prevention (CDC), and its sensitivity and specificity have been estimated to be 97 per cent and 99 per cent respectively.17,18 This assay has also been used in regional reference laboratories by the Pan American Health Organisation (PAHO) for confirmatory testing of all sera positive or indeterminate by commercial indirect IgM measles assays in screening laboratories, as well as a 10 per cent random sample of negative sera.6 A reference laboratory network is currently being established in Australia to provide confirmatory measles testing and serological quality assurance.
1.2.3. Alternative methods of diagnosis
Serodiagnosis may also be made by demonstrating IgG seroconversion (change from negative to positive) or rise in measles specific IgG antibodies. Measles specific IgG generally peaks approximately two weeks after onset of rash.19 Paired sera are collected 10 to 30 days apart, the first of which should be sampled in the week following rash onset, and the sera are tested simultaneously. For reasons of convenience and timeliness, IgG testing is not recommended for routine measles diagnosis, but is necessary for measuring population susceptibility.A variety of methods are available for detection of measles IgG or total antibody. Plaque reduction neutralisation (PRN) is the gold standard assay for determining protective immunity to measles,20 although measles specific antibody detectable by any test has been considered to represent immunity.21 Quantitative assays such as immunofluorescent assays, neutralisation, haemagglutination inhibition (HAI), complement fixation tests (CFT) and PRN, may be used to demonstrate four fold rises in measles antibody, unlike EIA which is a semi-quantitative assay, and cannot be routinely used in this manner.22 CFT are no longer recommended for measles diagnosis, and HAI is known to have inferior sensitivity compared to more modern assays.19
1.2.4. Serodiagnosis following immunisation
Following measles immunisation, seroconversion usually occurs, and measles specific IgM may be detected for one to two months. Serologically diagnosed cases who received a measles containing vaccine 6-45 days prior to testing should be classified as confirmed measles only if they are also linked epidemiologically to another confirmed case.14 Viral culture and molecular methods can distinguish between vaccine virus and wild strains.23Top of page
1.2.5. Viral culture and molecular epidemiology
Viral culture is not currently recommended for routine diagnosis of acute measles. However, characterisation of measles isolates will become important in discerning whether future measles outbreaks are caused by strains of domestic origin - which implies failure to interrupt local transmission - or by imported strains of measles.In the USA, molecular epidemiological analysis based on nucleotide sequencing of either haemagglutinin or nucleoprotein genes has been used together with standard epidemiological techniques to provide this capability. It appears that a single indigenous measles genotype was once prevalent in the USA. Now the situation is more heterogeneous, and an increasing proportion of cases are caused by measles strains previously seen largely in Japan, Europe, and Africa.24 Currently, eight genotypic groups of measles are known to be circulating worldwide.25 A global network and a standard system of genotype nomenclature is being developed to help track measles transmission world wide.
Characterisation of a representative sample of current and past Australian isolates is required prior to the vaccination campaign, to enable these powerful molecular epidemiological tools to be employed during the elimination phase.
When to collect specimens for culture
Specimens for culture should be collected from at least one case in every chain of measles transmission (2 or more epidemiologically linked cases), and from at least two cases during an outbreak investigation (Section 3.1.2). The yield from sporadic cases is likely to be low, because clinical diagnosis is unreliable in this setting. A nasopharyngeal aspirate is the specimen of choice for measles culture. Urine, heparinised blood and throat swabs are also suitable specimens. Culture should be performed simultaneously with initial serology, rather than waiting for serological confirmation, as measles virus is rarely shed for more than a few days after onset of rash. The virus may be present in respiratory secretions for up to one to two days after onset of rash,26 and in the urine for up to 10 days.27 Contact a reference laboratory regarding the best method of specimen collection and transportation before sending specimens for culture. All positive measles cultures must be referred for molecular typing.1.2.6. Salivary antibody testing
For diagnosis
It can be difficult to obtain serological confirmation for a large number of suspected measles cases, and considerable interest has been focussed on the possibility of convenient, non-invasive diagnosis of measles using salivary specimens. Saliva has been shown to contain measles specific IgM antibodies in greater than 90 per cent of cases where measles IgM is present in serum.16,28 Salivary measles IgM testing is now in routine use in measles surveillance in the United Kingdom, but not as yet in the USA.10 There are technical difficulties with serological tests of saliva, and currently these tests are not available for routine diagnosis of measles in Australia.For serological surveys
Salivary antibody tests have also been used for seroprevalence studies in paediatric populations. Unfortunately, salivary detection of measles IgG antibodies is very insensitive compared with their detection in serum, and it is unlikely that this method will be useful for population surveys of susceptibility.291.2.7. Differential diagnosis
Several other infectious diseases can mimic measles, and when measles is well controlled, the majority of suspected cases have alternative aetiologies. The most common of these are: Human herpes virus 6 (exanthem subitum), rubella, enterovirus, and Human parvovirus B19.10 In cases of suspected measles which are rejected on the basis of serological testing, it is recommended to test for rubella, and other diseases as clinically indicated. Measles reference laboratories will intermittently measure prevalent causes of rash illness by cross-sectionally testing negative sera for a variety of pathogens. This will provide supportive evidence for measles elimination in later stages of the campaign.1.3. Case investigation
All cases (suspected and confirmed)
Following a report of suspected measles, clinical information needs to be collected to establish whether a notified case meets the clinical case definition described in Section 1.1.1. As soon as possible after notification, collect serum for testing on all suspected cases, and specimens for culture where indicated (see section 1.2.5).It is important to collect accurate and complete immunisation histories on all cases, including the number of doses and dates when measles-containing vaccines were given. Wherever possible, documentation of vaccination should be sought from written records or registers. This may be difficult for teenagers and adults, for whom self report may be the only available source of information. Document the source of immunisation information on the data collection form (Appendix A).
Collecting demographic data helps characterise cases and detect temporal or geographic clustering of cases. Monitoring disease outcomes, such as death and encephalitis is also important, because the main purpose of measles control is to prevent severe illness and death. Enhanced surveillance is likely to increase notifications of suspected measles, but an increasing proportion of these may be mild or modified by prior vaccination.
Look for the source of infection in all cases of measles. When no apparent history of exposure exists, look for situations where unrecognised exposure may have occurred, such as: day care, school, air travel, indoor sporting events, and contact with overseas visitors.
Appendix A is a sample form which summarises the core data that should be collected during case investigation. These data will be collected and collated at a national level, but additional data will be required for individual case follow up and evaluation of surveillance at a local level, including: the identifying data for reporting authorities, doctors and laboratories, affected institutions, and contacts; dates of laboratory specimen reception and reporting.
Confirmed and epidemiologically linked cases
Identify contacts, establish their immunisation status, and assess the potential for further transmission. Contacts are persons who have been exposed, for any length of time, to a laboratory confirmed or epidemiologically linked case during their infectious period (4 days before to 4 days after rash onset); exposure must be face-to-face or in a confined setting such as a class room. Measles is highly infectious and brief exposure can result in infection. Transmission is most likely to occur in confined settings and institutions, and to those without documented vaccination. Contacts aged 12 months to four years should receive measles-mumps-rubella (MMR) vaccination if they do not have documented evidence of prior vaccination. Contacts aged 5 years and over who are attending primary and secondary schools should be vaccinated with MMR if they are not up to date with the new MMR schedule - that is, have not received two doses of a measles containing vaccine. Contacts should be vaccinated within 72 hours of exposure. Vaccination is not harmful if given later, but it is unlikely to prevent infection.Refer to the NHMRC document Measles: guidelines for the control of outbreaks in Australia for current recommendations regarding: the use of normal human immunoglobulin in contacts who are immunosuppressed or aged less than 12 months; vaccination of high risk populations such as Northern Territory Aboriginals; exclusion of cases and contacts.1
Top of page
1.4. Data flow, analysis and reporting
Notification data should be forwarded weekly to State authorities, and fortnightly to the National Centre for Disease Control. Case investigation data for both suspected and confirmed cases should be forwarded for State and national collation.Notification data should be reviewed daily at a local level, and fortnightly nationally. Data should be presented by age, sex, vaccination status, and locality at the local government area (by States), and by State and Statistical Division nationally. Data analysis and interpretation should be disseminated at State and national levels at least fortnightly, preferably in a dedicated measles control report.
2. Enhancing surveillance
Existing state-based disease notification systems - which rely primarily upon unsolicited reports from doctors, laboratories, and hospitals - provide a sound basis for enhanced measles surveillance. However, enhancing surveillance through additional case finding is required for successful measles elimination.2.1. Improving case ascertainment
New cases must be notified by telephone to the local or State/Territory health authority, and an attempt must be made to confirm the diagnosis within 24 hours of notification. Case investigation will help identify source cases and subsequent transmission to other settings. Additional cases must be sought intensively and notified separately. In this way, a chain of measles transmission must be pursued as far as possible. For sporadic cases this will usually involve interviewing: the person who notified the case, the case or one of their family members and the case's school or workplace. As a rule of thumb, seek additional cases with rash onset three weeks before and after that of the index case.2.2. Active surveillance
Active surveillance is the process of seeking measles cases other than through routine unsolicited reports. It should be used to evaluate, stimulate, and hasten routine surveillance mechanisms where deficiencies are expected, for example in areas of low vaccination coverage and low measles incidence. Active surveillance can involve contacting schools, doctors, laboratories, and hospitals, seeking cases that have not already been notified. Reviewing additional disease registers or data sets which are not analysed routinely - such as emergency department and laboratory registers - can help determine the magnitude, geographic extent, and beginning and end of outbreaks. Case finding methods need to be tailored to local health services and surveillance objectives. For example, determining the extent of a measles outbreak in a remote community will require a different approach to evaluating the sensitivity of passive surveillance for hospital admissions in an urban health area. In view of the measles vaccination campaign, by July 1998 local health authorities must review mechanisms for quickly instituting active surveillance for measles via local laboratories and health services, and in local communities and institutions at high risk for measles outbreaks.Alternative data sources
Inpatient statistics and mortality data provide valuable alternatives for examining secular trends in rates of severe disease. These data may be less affected by ascertainment bias than notifications. However, medical and administrative staff of hospitals must ensure that cases admitted for treatment of measles complications, have measles mentioned in the admission and discharge diagnoses. These data sets should be examined and compared to disease notification data at least annually. In addition, where identifying fields are available, cross checking these data against measles notifications can identify deficiencies in the completeness of case ascertainment and outcome monitoring.2.3. Monitoring surveillance quality
There is no single disease control indicator for measles - such as acute flaccid paralysis for poliomyelitis - which allows an independent means of monitoring measles control. Therefore, quality assurance is operational, rather than validating using an alternative measure for measles incidence. The following will be used as key operational indicators of measles surveillance quality:- The proportion of all cases that are subjected to laboratory testing for measles;
- The median time from rash onset to specimen collection;
- The median time from specimen collection to notification of the local/State health authority; and
- Percentage of cases with data on immunisation status.
3. Outbreak investigation
Monitoring and investigating measles outbreaks provides valuable information for control initiatives, and helps strengthen surveillance. Outbreak investigations help characterise populations at risk, and may be used to answer specific research questions. They provide an excellent opportunity to measure vaccine effectiveness, and to evaluate new diagnostic methods.30,31 A full description of an approach to outbreak investigation is beyond the scope of this document, and only a framework is provided.3.1.1. Outbreak definition
Two or more laboratory confirmed cases which are related in time and place, or a single laboratory confirmed case in an institution (e.g. school).As a rule of thumb, cases are considered related in time if the serial interval (time from rash onset in the first to rash onset in the second) is three weeks or less. As we move to towards elimination every confirmed measles case should be considered an outbreak.
Top of page
3.1.2. Outbreak investigation
When clusters of suspected measles occur, an attempt should be made to obtain serological confirmation, and samples for culture, on at least two cases. For confirmed measles cases, the standard case investigation form can be used, but it may not be possible to complete these data for all suspected cases.A minimum outbreak investigation would:
- Ascertain age and immunisation status for all suspected cases;
- Assign a unique outbreak name or number to help identify the cases which form part of an outbreak (Appendix A);
- Complete the data collection form for the index case and at least two confirmed cases; and
- Estimate age-specific immunisation coverage for the population/region affected by the outbreak. These data may be extracted from immunisation registers, by examining data from previous surveys, or by performing a new survey.
3.1.3. Monitoring outbreaks
Collecting outbreak investigation data in this way will allow outbreaks to be evaluated in more detail using surveillance data. The regional frequency of outbreaks will be compared - a dot map showing the distribution of outbreaks by health area is a helpful way to present these data. The interval between outbreaks may also be examined by region, and can be used to anticipate the timing of outbreaks.Performance indicators will also be used to monitor the quality of outbreak investigations. For example, the proportion of outbreak cases with vaccination data; and the proportion of outbreak investigations where at least one specimen was submitted for viral culture.
4. Monitoring vaccination coverage and population susceptibility
Measuring vaccination coverage and population susceptibility determines whether control targets are being reached, and helps predict outbreaks and plan vaccination strategies.4.1. Vaccination coverage
Vaccination coverage is a key indicator of campaign success and predicts measles control. The following are some important principles regarding vaccination coverage monitoring in the setting of a measles elimination initiative.4.1.1. Monitoring the routine immunisation schedule
The Australian Childhood Immunisation Register (ACIR) is now yielding quarterly reports for measles coverage on cohorts of 2 year old children who were born since the ACIR commenced in January 1996. These coverage reports are presented by State, but similar tabulations will be used to report data to the level of local government area for use by local immunisation program managers. Routine performance indicators are currently being developed to monitor the quality of ACIR coverage data. In addition, a mechanism is being developed to quickly identify regions or providers that are not achieving coverage targets, so that appropriate improvements can be planned.At present data are scanty regarding coverage with the second dose of MMR. When the second dose of measles vaccination is brought forward and is given to preschool children instead of adolescents, this dose will also be monitored using the ACIR.
In addition, surrogate measures of coverage, such as vaccine distribution, should be monitored. This will aid interpretation of trends in ACIR data during the initial years of its operation, when apparent improvements in coverage may actually represent improved participation. Intermittent cross-sectional surveys will also be used to validate ACIR coverage data. Coverage should also be measured during outbreak investigations (see section 3.1.2).
Top of page
4.1.2. Mass vaccination campaign
Vaccination registers are not suitable for measuring coverage during the school based campaign. The ACIR collects data only for children under 7 years of age, so tally sheets will be used by school vaccination teams to count vaccine doses versus students enrolled. Preschool doses, given by the child's usual provider, will be measured using the ACIR.4.2. Measles susceptibility
As measles is controlled and fewer cases occur, estimates of population susceptibility obtained from serological surveys become an increasingly important source of information regarding the success of the measles elimination program. The National Centre for Immunisation Research and Surveillance of Vaccine Preventable Diseases (NCIRS) plans to conduct regular serological surveys every two to three years for persons aged 2 to 60 years. These regular serosurveys will be conducted by testing serum residues from blood samples which are referred routinely to major public health laboratories in all States and Territories. These sera will be tested for a range of vaccine preventable diseases including measles and rubella. Blood samples referred from immunosuppressed persons will be excluded.This serological surveillance will help evaluate the effects of moving the second dose of MMR from adolescence to preschool. It will allow us to monitor changes in measles susceptibility, and confirm that the prevalence of rubella susceptibility remains low in women of child bearing age. Susceptibility data can also be used in conjunction with mathematical modelling to predict the expected timing, size, morbidity, mortality, and age distribution of outbreaks.32,33 Serological surveillance has been used routinely in Britain for the past 10 years, and using these data it was predicted that a large measles outbreak would occur in Britain in 1994.34 A mass vaccination campaign of school children was implemented in response to this, and it appears that the expected outbreak has been successfully prevented or delayed.10,35,36 More recently, it was predicted that a measles outbreak would occur in New Zealand during the years 1997-98, and an outbreak did occur in early 1997.37
5. Monitoring vaccine safety and effectiveness
5.1. Vaccine safety
The MMR vaccine licensed in Australia has an excellent safety record. Fever, occurring 6 to11 days after vaccination is the most commonly reported adverse event.38 However, the majority of persons in catch-up campaigns are already immune to measles, and consequently vaccine virus related adverse event rates (AEs) are usually lower than for vaccination at 12 months of age.5 Despite this, because catch-up campaigns are necessarily well publicised and a large number of vaccinations are administered over a short period of time, the absolute number of events in any reporting period is increased. As a result, public anxiety regarding AEs is often heightened during mass campaigns.39In order to maintain public confidence, adverse events to vaccines used in mass vaccination campaigns should be given a high priority. It is important to inform doctors and measles campaign staff regarding possible AEs, and remind doctors regarding the importance of AE reporting. A detailed description of the adverse events associated with MMR vaccination is available in the 6th Edition of the Australian Immunisation Handbook.2 Reports of adverse events should be made to the State/Territory health departments, or to measles campaign staff. Providing a 24 hour telephone hot-line may also improve the timeliness of AE reports and public confidence. However, the staff supporting such services must be well briefed on recent controversies regarding MMR vaccine safety, and capable of fielding AE reports or referring them appropriately.
During the mass vaccination campaign, State/Territory AE reports, including outcomes of serious events such as convulsions, should be updated daily and sent to the State or Territory vaccination team. For the routine schedule, AE rates will be calculated using the number of vaccinations reported to the ACIR as the denominator. During mass campaigns, vaccination tallies collected by the vaccination teams will be used for this purpose. Background national rates for some of the diseases which may be confused with vaccine related events - such as encephalitis and Guillain-Barr� syndrome - can be estimated using alternative data sources such as inpatient statistics data and surveillance for acute flaccid paralysis. These comparative data will be useful during the vaccination campaign, to evaluate whether reporting rates during the campaign differ from pre-existing rates.
5.2. Vaccine effectiveness
In the future, when more accurate coverage data are available and vaccination status is collected for measles notifications, surveillance data will be used to monitor measles vaccine effectiveness (VE).40 Accurate coverage statistics are needed, because small changes in coverage can markedly influence calculations of VE using the 'screening' method. Coverage data, must also reflect the populations and age groups from which notification data originate. Notification biases influence screening estimates of VE, so trends will be more reliable than absolute values. Outbreak investigations can also be used to evaluate measles vaccine effectiveness.315.3. Cold chain monitoring
Monitoring the cold chain is an important quality control measure which cannot be addressed adequately in this surveillance plan. Guidelines for transport and storage of vaccines are outlined in the Australian Immunisation Handbook.2 MMR vaccine is distributed as a freeze dried preparation, and prior to reconstitution it is relatively resistant to fluctuations in temperature. Data regarding the adequacy of MMR vaccine storage and transport do not need to be collated and analysed nationally.Top of page
Conclusion
This strategy recommends numerous surveillance enhancements that are required to support a measles elimination initiative in Australia. The key elements of this strategy are:- Revised control targets both for measles vaccination coverage and population susceptibility (page 42).
- Uniform, simple, and sensitive measles case definitions; including a definition for imported infection (Section 1.1).
- Pursuing serological testing (IgM) for all suspected measles cases; and referral of all positive sera from sporadic cases to a reference laboratory for confirmation (Sections 1.2 and 1.3).
- Collecting specimens for culture from at least two cases in a measles outbreak, and referring all positive cultures for molecular typing (Section 1.2.5).
- Uniform case investigation, and (minimum) data collection which includes vaccination status for all notifications (Section 1.3).
- The use of active surveillance to evaluate and enhance routine surveillance mechanisms (Section 2.2).
- The use of standard indicators to monitor the quality of surveillance data (Section 2.3).
- Investigation of all measles outbreaks, collecting uniform (minimum) data regarding the outbreak (Section 3).
- Enhancing surveillance of adverse events following immunisation (Section 5.1).
- National serological surveys to monitor the effectiveness of the measles immunisation program and the effects of changes to the MMR vaccination schedule.
Top of page
Acknowledgements
The following persons contributed to the development of this strategy: Dr Osman Mansoor (New Zealand Ministry of Health); Dr Mahomed Patel (National Centre for Epidemiology and Population Health); Dr Bronwen Harvey, Mr Ross Andrews, and Ms Sue Campbell Lloyd (National Centre for Disease Control); Dr Robert Hall (SA Health), Dr Linda Selvey and Dr Gerard Neville (Queensland Health); Dr Mark Ferson, Dr Margaret Ashwell, and Dr Jeremy McAnulty (NSW Health); Dr Jag Gill and Dr Tony Watson (WA Health); Dr Graham Rouch, Dr John Carnie, and Dr Rosemary Lester (VIC Health), Dr Avner Misrachi (TAS Health), Dr Angela Merianos (NT Health); and Ms Irene Passaris (ACT Health).Top of page
References
1. National Health and Medical Research Council. Measles: Guidelines for the control of outbreaks in Australia, 1996.2. National Health and Medical Research Council. The Australian immunisation handbook: Chapter 3.4 - Measles Mumps Rubella. 1997; Sixth Edition: 82-96.
3. Expanded Programme on Immunisation. Using surveillance data and outbreak investigations to strengthen measles immunization programmes. 1996; 96.02: 1-24.
4. Murray CJL, Lopez AD. Evidence-based health policy - lessons from the Global Burden of Disease Study. Science 1996;274: 740-743.
5. National Advisory Committee on Immunisation (NACI). Supplementary statement on measles elimination in Canada. Canada Communicable Diseases Report 1996;22:9-15.
6. Anonymous. Measles eradication: recommendations from a meeting cosponsored by the World Health Organization, The Pan American Health Organization, and the Centers for Disease Control. MMWR 1997;46: 1-20.
7. Measles - United States, 1996, and the interruption of indigenous transmission. MMWR 1997;46: 242-246.
8. Accelerated surveillance and control of measles in the Western Pacific Region. 1996; WPR/VID/EPI (2)96.8: 1-13.
9. Ferson MF, Young LC, Robertson PW, Whybin LR. Difficulties in clinical diagnosis of measles: proposal for a modified clinical case definition. Med J Aust 1995;163: 364-366.
10. Gay N, Ramsay M, Hesketh L, Morgan-Capner P, Brown D, Miller E. The epidemiology of measles in England and Wales since the 1994 vaccination campaign. Communicable Disease Report 1997;7: R17-21.
11. Benenson AS, Chin J. Measles. In: Control of Communicable Diseases Manual, 16th ed. Washington: American Public Health Association, 1995: 293-298.
12. Vitek CR, Redd SC, Redd SB, Hadler SC. Trends in importation of measles to the United States, 1986-94. JAMA 1997;277: 1952-1956.
13. Anonymous. Measles - United States, 1997. MMWR 1998;47: 273-276.
14. Centers for Disease Control and Prevention. Chapter 6: Measles. In: Manual for the surveillance of vaccine-preventable diseases. Centers for Disease Control and Prevention, Atlanta, GA, 1996.
15. Mayo DR, Brennan T, Cormier DP, Hadler J, Lamb P. Evaluation of a commercial measles virus immunoglobulin M enzyme immunoassay. J Clin Microbiol 1991;29:2865-2867.
16. Helfand RF, Heath JL, Anderson LJ, Maes RF, Guris D, Bellini WB. Diagnosis of measles with an IgM capture EIA: the optimal timing of specimen collection after rash onset. Journal of Infectious Diseases 1997; 175: 195-199.
17. Erdman D, Anderson L, Adams D, Stewart J, Markowitz L, Bellini W. Evaluation of monoclonal-based capture immunoassay for detection of specific antibodies to measles virus. J Clin Microbiol 1991;29: 1466-1471.
18. Hummel K, Erdman D, Heath B, Bellini W. Baculovirus expression of the nucleoprotein gene of measles virus and utility of the recombinant protein in diagnostic enzyme immunoassays. J Clin Microbiol 1992;30:2874-2880.
19. Griffin D, Bellini W. Measles virus. In: Fields BN, Knipe DM, Howley PM, eds. Fields Virology, 3rd ed. Philadelphia: Lippincott-Raven, 1996.
20. Chen RT, Markowitz L, Albrecht P, et al. Measles antibody: reevaluation of protective titres. J Infect Dis 1990;162:1036-1042.
21. Anonymous. Measles prevention. MMWR 1989;Suppl9:38:1-18:1-18.
22. Duncan R. The use of ELISA for rapid diagnosis: antibody detection. In: Kemeny D, Challacombe S, eds. ELISA and other solid phase immunoassays, Wiley and Sons, 1998.
23. Mori T. A simple method for genetic differentiation of the AIK-C vaccine strain from wild strains of measles virus. Biologicals 1994;22:179-185.
24. Rota JS, Heath JL, Rota PA, et al. Molecular epidemiology of measles virus: identification of pathways of transmission and implications for measles elimination. Journal of Infectious Diseases 1996;173: 32-37.
25. Anonymous. Progress towards global measles control and elimination, 1990-1996. MMWR 1997;46: 893-897.
26. Ruckle G, Rogers K. Studies with measles virus: II: isolation of virus and immunological studies in persons who have had the natural disease. J Immunol 1957; 78: 341-355.
27. Gresser I, Katz SL. Isolation of measles virus from urine. N Engl J Med 1960;275: 516-523.
28. Brown DW, Ramsay ME, Richards AF, Miller E. Salivary diagnosis of measles: a study of notified cases in the United Kingdom, 1991-3. BMJ 1994;308: 1015-1017.
29. Cutts FT, Bartoloni A, Guglielmetti P, Brown D, Bianchi Bandinelli ML, Roselli M. Prevalence of measles antibody among children under 15 years of age in Santa Cruz, Bolivia: implications for vaccination strategies. Transactions of the Royal Society of Tropical Medicine and Hygiene 1995; 89: 119-122.
30. Gustsafon TL, Lievens AW, Brunell PA, et al. Measles outbreak in a fully immunised secondary-school population. N Engl J Med 1987; 316: 771-774.
31. McDonnell LF, Jorm LR, Patel MS. Measles outbreak in western Sydney. Vaccine failure or failure to vaccinate? Med J Aust 1995;162: 471-475.
32. Babad HR, Nokes DJ, Gay NJ, Miller E, Morgan-Capner P, Anderson RM. Predicting the impact of measles vaccination in England and Wales: model validation and analysis of policy options [published erratum appears in Epidemiol Infect 1995 Aug;115(1):213]. Epidemiology and Infection 1995;114:319-344.
33. Gay NJ, Hesketh LM, Morgan-Capner P, Miller E. Interpretation of serological surveillance data for measles using mathematical models: implications for vaccine strategy. Epidemiology and Infection 1995;115:139-156.
34. Morgan-Capner P, Wright J, Miller CL, Miller E. Surveillance of antibody to measles, mumps, and rubella by age. BMJ 1988;297:770-772.
35. Gay NJ, Miller E. Was a measles epidemic imminent? Communicable Disease Report CDR Review. 1995;5:R204-207.
36. Ramsay M, Gay N, Miller E, et al. The epidemiology of measles in England and Wales: rationale for the 1994 national vaccination campaign. Communicable Disease Report CDR Review 1994;4:R141-146.
37. Tobias M, Mansoor O. Predicting the next measles epidemic. NZ Public Health Rep 1997;4: 1-3.
38. Anonymous. Update: vaccine side effects, adverse reactions, contraindications, and precautions. Recommendations of the Advisory Committee on Immunization Practices (ACIP). MMWR 1996;45:1-35.
39. Salisbury DM, Campbell H, Edwards B. Measles Rubella Campaign in England. One year on. Health Promotion Division and Measles Control Agency, Department of Health, November 1995.
40. Orenstein WA, Bernier RH, Hinman AR. Assessing vaccine efficacy in the field. Further observations. Epidemiol Rev 1988;10: 212-241.
Appendix A. Measles Data Collection Form
Editor's note: Appendix A is available in the hard copy of CDI but is not available as an HTML on the Internet. Please contact us if you wish to obtain a copy.This article was published in Communicable Diseases Intelligence Volume 23, No 2, 18 February 1999.
Chapter 1
eISBN: 978-1-78262-677-0
From Book Series: Chemical Biology
David Murraya and Mark Wigglesworth*a
a Discovery Sciences, Global HTS Centre, AstraZeneca, Alderley Park, SK10 4TG, UK. E-mail: mark.wigglesworth@astrazeneca.com
High throughput screening (HTS) remains the key methodology for finding hit and lead compounds within the pharmaceutical industry and also, recently, in the academic drug discovery community. HTS has changed significantly in AstraZeneca over the last 15–20 years with a massive expansion of the number of compounds available to screen, increasing industrialisation and automation of the process in order to cope with larger numbers of compounds, and more recently, the running of screens from external collaborators via open innovation initiatives. In this chapter we will discuss how this approach to HTS has been developed within AstraZeneca and pay particular attention to how the optimisation and validation of the wide spectrum of assays that we have to deal with in our group is done. We will discuss how we accept assays into HTS from our assay development groups and describe how these assays are validated and optimised for use as an HTS screen.
1.1 Introduction
High throughput screening (HTS) remains the key methodology for finding hit and lead compounds within the pharmaceutical industry1 and also, recently, in the academic drug discovery community. HTS has changed significantly in AstraZeneca over the last 15–20 years with a massive expansion of the number of compounds available to screen, increasing industrialisation and automation of the process to cope with larger numbers of compounds, and more recently, the running of screens from external collaborators via open innovation initiatives. It has also become evident over the last 5 or so years (at least in AstraZeneca) that a more nuanced approach to HTS is required, where a large repertoire of assays is needed that spans from very high throughput “industrial” biochemical assays for targets such as kinases to highly complex cell based phenotypic assays2 on hard to source cells such as primary cells, genetically engineered human cell lines and induced pluripotent stem cells. We are also using a wider range of detection methods, from standard plate reader assays through to technologies such as flow cytometry, imaging and high throughput mass spectrometry. This presents very significant challenges in designing and developing complex cell and biochemical assays for the assay development teams, and huge challenges to the HTS group to run hundreds of thousands to millions of compounds through these assays.
There are perhaps two core models of how to run HTS in drug discovery. The simplest and arguably most efficient model is to limit the repertoire of assays to very few detection technologies, and if the assay cannot run in this mode it will not be run. This allows both operational and cost efficiencies, and increases the productivity of a limited team. However, this model can also limit the impact of HTS on drug discovery by narrowing the targets that undergo HTS. Within AstraZeneca we run a model of HTS where we will try and run complex biochemical or cell based assay as high throughput screens. This promises to find a wider range of hits against a wider range of targets but it does require very sophisticated and costly automation platforms and considerable effort is needed to develop assays robust enough to screen large compound libraries. This requires staff with a wide range of experience and expertise. We need people with experience in running large scale assays and the management of the logistics of such assays. We also require experts in automation, informatics and statistics plus more specialised technologies such as flow cytometry and mass spectrometry. We also need to mirror some, but not all, of this expertise in the assay development teams. This makes staffing of such an HTS department more difficult, and with a need for more specialisation, departments can become less flexible.
In this chapter we will discuss how this approach to HTS has been developed within AstraZeneca and pay particular attention to how the optimisation and validation of the wide spectrum of assays that we have to deal with in our group is done. We will discuss how we accept assays into HTS from our assay development groups and describe how these assays are validated and optimised for use as an HTS screen.
1.2 HTS at AstraZeneca
Within AstraZeneca we have a single global HTS centre that provides high throughput screening for all AstraZeneca disease areas as well as our collaborators who have taken advantage of the various open innovation initiatives that AstraZeneca has launched. The HTS centre sits in an organisation within AstraZeneca called Discovery Sciences. Discovery Sciences supplies a large set of scientific and technical services to AstraZeneca, allowing for consolidation of the expertise and infrastructure to supply these vital components of the drug discovery value chain. This results in the HTS group interacting widely across the business as well as outside of it. In terms of reagent supply and assay development of high throughput screens, this is carried out by a separate group within Discovery Sciences called Reagents and Assay Development (RAD). Although introducing a handover step into the HTS process, this again allows the consolidation of expertise and infrastructure to both save cost and increase quality. However, the handover does present challenges to both the assay development and HTS groups, who must make sure that all assays required for HTS are of the quality that is required to support the costly undertaking of a screen. This organisational structure has led to a considered process of defining the criteria for an acceptable screen, accepting the screen and validating the screening assay to ensure that it is indeed suitable for an HTS campaign without incurring a large bureaucratic burden. Although some have questioned the need for these criteria, it is our experience that the standards defined within them are vital to facilitate the transfer and deployment of successful screening assays. Without this foundation we have found that standards inevitably slip and different practices spring up within and across groups, leading to issues with assays of varying quality being prepared for HTS.
1.2.1 Criteria and Acceptance
HTS is both costly to set up, with a high initial capital outlay, and a demanding process to maintain and run, yet it remains a good return on investment by being the most productive hit finding strategy we employ. To be able to screen millions of compounds and get a set of reliable data is difficult. Equally, HTS is the main method for finding novel chemistry for projects within AstraZeneca and beyond, and it is critical for keeping the pipeline of drug discovery projects filled with high quality chemical equity. Within HTS we have developed a set of criteria that will result in assays that are fit for the task of finding chemical leads.
However, it is worth reiterating that these are not hard and fast rules. What is important is that these guide the scientists to have a conversation regarding what risks are acceptable, where the problems lie and how they can be overcome. It would be our advice to anyone looking at these criteria to assess the quality of assays as early as possible as this will minimise the possibility of re-work later in assay development. These “mini” validation experiments combined with the recommended statistics can really help to define why and how an assay needs to be modified to become a good HTS assay.
The overriding aim is the development of robust assays. In many respects HTS is an anomaly in drug discovery in that the vast majority of data are generated by taking a single concentration of a compound and testing it just once in an attempt to see if it is active against a biological target. Of course there is an element of replicate testing in large HTS collections as there are clusters of compounds of similar structures, but an HTS assay needs to be sensitive enough to detect relatively weak compounds and robust enough that the false positive and negative rates are low. Much focus is on false negatives, as of course we do not like to think we have missed something. However, managing false positives is, arguably, a greater challenge and can lead to compounds being missed as teams try to separate true hits from many hundreds or thousands of false hits. Additionally, the assay has to be of a form that can actually be run on the automation platforms we have or be run on manual workstations at a throughput that allows the assay to complete in the time frame required to allow the flow of projects through a portfolio of assays. An assay also has to be reliable in that if it is run twice it will find the majority of active compounds both times, confirming that the hits are not due to random events. It quickly becomes apparent that there are some key criteria that an HTS assay has to fulfil to maximise its utility in finding hit compounds: Robustness Reliability As simple to run as possible Affordable Relevant
In this discussion we will focus on the first three bullet points in explaining how we have generated a set of criteria to help design good HTS assays. Affordable is a given in many respects in that an assay has to fit within a budget. We do run assays with quite a range of different costs but there always has to be a balance between the cost and maximising both how easy the assay is to run and the ability to find hit compounds. Relevant is a key criterion and may seem obvious but is worth stating. The assay has to be relevant to the biological or disease process that we are wishing to disrupt or stimulate. Anything other than this wastes the investment in the screen. Robustness and reliability in many respects overlap, and in fact, robustness should lead to reliability.
1.2.2 Robustness/Reliability
Within HTS at AstraZeneca robustness of an assay is key. In our experience, a lack of robustness is the key reason we will struggle with an assay or in extreme circumstances stop the assay running. Determining robustness is a large topic with many differing opinions. We will discuss what works for us and it should be noted that in many of these topics another criterion we use is to keep things simple and understandable for the scientists doing the screening (and indeed the assay developers) whilst having a fit for purpose set of criteria. In Figure 1.1 we give the criteria that we use when setting out to develop an HTS assay. These are an attempt to generate robust and reliable assays that will pass assay validation. They are derived from our experience across AstraZeneca and other Pharma companies in HTS over the last 15 years and are there to guide the user to make informed decisions rather than being a simplistic check list. They are by no means an exhaustive list but are what we consider to be key. Below we look at some of these criteria one by one.
Z′-factor is a widely used parameter to help determine the robustness of an assay and its use for single shot screening. It is simple to understand and is popular across assay development and screening groups due to its proven utility. We use the robust Z′-factor to determine how sensitive the assay will be in finding hits and as a measure of the robustness of the assay from the performance of the control wells. We do not use Z-factor routinely (although it is calculated in our data analysis package) as we screen focussed libraries of compounds, which can be an issue because the very high hit rates result in a compound activity distribution that does not define the true central reference for the assay, leading to an artificially low Z-factor. Additionally, sticking to the Z′-factor gives consistency across the assay development and HTS groups when comparing data. The reason why we have adopted the robust Z′-factor {where standard deviation is replaced by robust standard deviation [median absolute deviation (MAD)×1.483] and mean by median in the equation derived by Zhang et al.3} is to remove the influence of outliers on the Z′-factor and to remove the need for human intervention, which can result in people chasing a target Z′-factor value with subjective removal of “outlier” data. Although Zhang et al.3 state that assays can be used with a Z/Z′-factor as low as 0 to give a yes/no answer for an HTS primary screen, our experience has shown us that assays need to have a robust Z′-factor of at least ≥0.5 to perform robustly and reliably. We of course remain pragmatic and will take assays with a lower Z′-factor when the target is very high value and there is no alternative assay and/or nothing more can be done to improve an assay. In these circumstances we will look at other approaches to improve robustness such as replication in the assay, which almost certainly will reduce the number of compounds screened, or perform a quantitative HTS (qHTS) where concentration responses are run as a primary screen, again on a significantly reduced number of compounds.
A signal to background ratio (S : B) of >3 is used to ensure robustness and our experience again shows us that a relatively poor robust Z′-factor and a small S : B most likely will result in a poor assay unsuitable for HTS. This may again seem obvious but there is pressure from project teams to run assays, after all not running an assay guarantees not finding hits, and without a clear set of criteria clear decisions are harder to achieve. It is important that assay developers do not try and configure assay parameters solely to ensure the measurement of very high potency compounds to the detriment of a good S : B, especially as HTS most likely will not find such high potency compounds, and even if there were such compounds, an accurate measurement of potency at the HTS stage is not important; detection of active compounds is what we need.
Measuring the percentage coefficient of variation (%CV) across whole plates ensures that the dispensers and readers are functioning correctly, and if they are available, running known pharmacological standards as concentration responses gives confidence that the assay will find active chemistry in a screen, displays the same rank order of potency expected and can reliably estimate the potency of compounds. This in itself does not test the reliability of the primary single shot assay but is the foundation of a reliable assay. It is also important during screening to give confidence that assay sensitivity remains acceptable throughout an extended screening run.
Our assay development groups also run what we call a mini-validation set to test the reliability of the assay in detecting hit compounds. The mini-validation set is 1408 compounds from our main validation set (see Section 1.1.2.5) on both 1536 and 384 plates. Although it does not always contain hit compounds against all targets it is a useful set to run in that it does not take much effort, does not use too many precious reagents and will quickly flag issues such as high hit rates or poor reproducibility. The full validation set could of course be used at this stage but as we move to more complex screens with expensive and sometimes hard to resource reagents it is usually prudent to use the mini-validation set to preserve these reagents. With these data we can determine some simple parameters and assess the data to determine whether the assay is suitable for hit finding and can be moved to the HTS group for full validation and subsequent transfer. In Table 1.1 we show how the mini-validation data are used to determine, in this case, the screening concentration to be used. In the case of this epigenetic target, we expect a low real hit rate and a high artefact hit rate and the mini-validation data nicely show how we can determine key parameters for the screen at an early stage in the assay transfer. Additionally, we can use the output of the mini-validation exercise to determine the efficacy of any downstream assay to successfully remove false hits and allow the identification of true hits.
Criteria | 10 µM Screening concentration | 30 µM Screening concentration |
---|---|---|
Robust Z′ of each plate | 0.6, 0.6 | 0.6, 0.6 |
Shape of distribution | Pass: tight central peak with long left hand tail | Fail: broad central peak with heavy left hand tail |
Median of compound wells (% effect) <10% | −1.93, 0.94 | −8.46, −14.85 |
Robust standard deviation of compound wells (% effect) <15% | 9.56, 9.89 | 20.36, 17.00 |
Hit rate at Q1−1.5×IQR <5% | 6.7%, 6.4% | 18.8%, 21.8% |
<5% of maximum/DMSO control wells show >50% effect | Pass | Pass |
<5% of minimum control wells show <50% effect | Pass | Pass |
No obvious plate patterns | Pass | Pass |
Predicted confirmation rate >50% ([#confirmed hits/(((#hits run=1)+(#hits run=2))/2)]×100) | 84% | 75% |
It is important that both the HTS and assay development groups use similar (ideally the same) equipment to remove any issues that can arise during assay transfer with different equipment that perform differently. We have experienced transfers taking longer than necessary when we have used different equipment across the groups and this leads to whitespace (a term we use to describe downtime) in the project whilst the differences are investigated and corrected. The criteria we apply to the mini-validation assessment are essentially the same as for a full validation and are listed in Figure 1.1. The hit rate and reproducibility are key at this stage. A high hit rate can be particularly problematic and we try to ensure that the full screening cascade is in place prior to transfer so that we can test the ability of the assays to remove false hits and confirm real hits. We also have a small library of problematic compound classes such as redox compounds, aggregators and thiol-reactives to probe the sensitivity of a target and its assay to such compounds, which are commonly referred to as pan-assay interference compounds (PAINS).4 This is a key step in checking the robustness of an assay and also helps us to understand what assays are needed to remove a high hit rate associated with a class or classes of PAINS. This early information allows us to test the validity of the screening cascade. Having this view early on is key to ensure that we can successfully transfer an assay, validate and run the HTS and successfully prosecute its output.
1.2.3 Analysing Data to Define Robustness/Reliability
Another issue that can have a significant effect on the robustness and reliability of an assay is that of plate patterns. Plate patterns are often seen as we move to higher density plate formats and lower assay volumes. Reasons for plate patterns are many and varied, and certainly not a topic for detailed discussion here. One of our criteria is the absence of plate patterns, but unfortunately all too often we have plate patterns and they cannot be removed by taking practical steps such as incubating cell assay plates at room temperature for a period of time before placing them in an incubator. We will accept assays with a plate pattern but only if it can be corrected by the algorithms in our data analysis software, Genedata Screener®.5 Genedata Screener contains sophisticated and proprietary statistical algorithms designed to remove plate patterns whilst preserving genuine actives by looking for consistent patterns across multiple plates. In an HTS group, having access to such correction algorithms is essential. Genedata Screener® is a commercial software package likely out of reach of small and/or academic HTS groups due its relatively high cost, but there are alternatives available for lower or no cost, such as the B Score algorithm or the R Score algorithm.6–8 Indeed, many different normalisation and pattern correction methods have been described in the literature,9 each with their advantages and disadvantages. These would need to be implemented as some form of software tool and are often too complex to be implemented in Excel, for example, but anyone skilled in the art of programming in R statistics should be able to build a simple application. Alternatively, there are a range of freely available software tools, some of which are described by Makarenkov et al.10,11 In our experience, the choice comes down to assessing acceptable performance on your data and ensuring that the scientists running the screen and data analysis at least understand the resulting output. The data analysis package used in HTS is also an important choice and it is worth writing a few words on why we chose Genedata Screener®.
Up until 2013, AstraZeneca tended to develop most of its data analysis software in-house, which resulted in very functional software designed to tightly integrate with our processes. We would try to incorporate current thinking around data analysis into our software but the problem we faced with in-house developed software was that once the software was finished the development team was disbanded and the software was not developed any further. We also suffered from many different software packages being used across the business, leading to poor interoperability and increased costs. In standardising our software package we have reduced overall costs, improved interoperability, but more importantly, have invested in software that incorporates the latest thinking in data analysis techniques. With Genedata Screener® being a commercial product it is also regularly updated to keep pace with new screening technologies, such as combination screening and Biacore. As the pace of change in HTS gets faster it is important to be able to respond to this change in both the experimental science you carry out and also in your data analysis approach.
1.2.4 As Simple to Run as Possible
Again, the importance of making an HTS assay as simple to run as possible seems like a very obvious statement to make, but is easy to overlook this when designing HTS assays or thinking about running a million compounds or more. It can seem like the best option is to utilise the investments made in automation at every step. However, it is our experience that automation is not always the best answer. If an assay comes to HTS in a 1536 format and has a low number of additions we will almost always try to run it manually. This is because of the time taken to validate the automation system and/or set up complex automation such that it can run a new screen. Although we are constantly looking for more efficient ways of doing this and have recently invested a significant amount in redesigning our future automation with this in mind, our historic experience tells us that removing the optimisation steps for automation by starting a suitable screen manually can mean faster progression and fewer assay failures. Hence, we are always asking ourselves, what is the best approach to screening for this assay, and can it be simplified to remove processes and increase screening efficiency? It is also worth noting that with some recent phenotypic screens we have run assays over very long time periods and with a large numbers of steps, which we simply could not have envisaged running manually. By selecting the correct automation and investing in making these systems work efficiently, they are indispensable to our process.
1.2.5 Assay Validation
Once an assay has fulfilled the key criteria and is assessed as suitable to transfer into HTS then the next step is to optimise and validate the assay. Optimisation is the process whereby we check the assay runs in our HTS laboratory and start to optimise it for running at the scale that is required for the screen. Again, having the same dispensers and readers as the assay development teams makes this process much faster. In this phase we determine the throughput of the assay (i.e. how many plates can be run per day or batch) and whether it will run on the chosen automation platform, and once those conditions have been set, whether the assay detects active chemistry reliably. This process we call validation and is the final check before deciding whether to commit to a full HTS. In order to validate assays we have designed a library of compounds selected from the full screening deck that represents the diversity of the compound collection that will be screened. This is important as we can then use this set to assess hit rates and plan the steps needed to mitigate high hit rates. The set consists of approximately 7000 compounds available in both 384 and 1536 plate formats. Furthermore, we have two sets or picks that position the compounds on different plates and wells across the two picks. This is designed to assess any positional effects on the data such as plate patterns or compound carry-over and any consequences this may have for detection of hits. As a minimum we have found running pick 1 and pick 2 on separate days is sufficient to assess the reliability and validity of the assay, but we often run both picks on both days to assess intra- and inter-day variability. Additionally, we also investigate the paradigm of running low molecular weight (LMW) compounds at a higher concentration at this point, having previously defined the concentration at which the main body of compounds will be run during the mini-validation steps described above. This gives us an opportunity to differentiate across our compound collection and ensure that the assay is capable of running the LMW subset at a high concentration with the aim of finding all progressable chemistries, as described elsewhere.12
In addition to running the validation plates, we also run a batch of compound plates from the compound collection that is the same size as the daily batch size we plan to run in the screen. We can then place a set of validation plates at the start and end of this batch of plates, allowing us to test, at the same time, the repeatability/reliability of the hit calling and the stability of the assay over the time it takes to run a batch of plates. Although this should confirm the stability of the assay and reagents that have already been determined in the assay development phase, we do find assays where this does not hold up when being automated. Assumptions can be expensive and time consuming to resolve in HTS. Hence, full batch sizes in the format that the HTS laboratory will run the screen are required to de-risk these assumptions.
The validation data are only useful if we can analyse and extract information from them to help with our decision regarding whether to proceed with the full HTS screen. We have made various attempts at analysing the data. In one incarnation of analysing the validation data we had a close collaboration with our statistics colleagues and came up with a complex mixture modelling algorithm looking at patterns of how close the individual replicates were and breaking these down into different populations by their variability. Using these data we could model assay cut-offs and make predictions of false positive and negative results by looking at the frequency of replicates falling either side of the cut-off compared with the other replicates and the average of the replicates. This gave us great insight into the nature of the data we were generating, but the data summaries were difficult for non-statisticians to interpret and understand. Furthermore, with the screeners struggling to understand the data, it made it very difficult to explain them to the drug project teams and so we decided to discontinue using the tool. This in no way says anything about the use of expert statistical input in HTS data analysis, but does say to us that the people running the screens and the teams receiving the output need to understand the methodology to accept the decisions made based on the data. Using the knowledge gained from the mixture modelling analysis of our validation data we have established a series of data plots in Tibco Spotfire™ to present and analyse the data to ensure that the key criterion of repeatability can be assessed. In designing this set of visualisations alongside the criteria in Figure 1.1, we wanted to ensure that the data were accessible and understandable and aided decision making. In the example plots in Figure 1.2 we show a Bland–Altman plot13 to compare two of the picks of the validation set, which helps to clearly display any shift in activity between runs and how repeatable the data are, assessment of hit rates at various cut-offs and box plots showing plate to plate variability. These and the other plots in the Spotfire template (two of which are also shown in Figure 1.2) provide a very visual assessment of the data and are great for allowing cross-group discussion and decisions to be made, led by the HTS screener, regarding the validation data. We do not quantify false negative or positive rates but use the visualisations to look for compounds exhibiting this activity and use the plots alongside the criteria values in Figure 1.1. In general, we find false negative and positive rates to be low and not of major concern at the validation stages. False hits due to interference with the assay technology or compound toxicity in cell assays are more of an issue and these are dealt with by well thought out counter assays in a well-designed and validated screening cascade.
1.3 Summary
The HTS group supports all disease areas in AstraZeneca and also screens from outside of AstraZeneca via our open innovation initiatives. We as a group do not apply any restrictions on the assays we will run as long as they are of a quality that can run at the scale of throughput that is required, we have the equipment needed or can source it, and they fit into our budget. This is what makes HTS such a fundamental and productive hit finding technology within AstraZeneca, but equally this gives us a great challenge in understanding and validating a wide range of assay types and technologies, and illustrates why clear criteria around assay performance are so important. Looking to the future, we perceive that we will be performing more complex cell based assays on rare cell types such as primary human cells. Our experience today tells us that phenotypic cell assays can be challenging in HTS and require a different approach to enable screening, and quite often we have to accept screens that are less robust than those we have accepted for a simpler assay. When considering the range of assays we see in HTS, it is only reasonable that our expectation of performance will be different and we expect assay performance criteria to evolve over time. However, we believe that the process we have in place both helps to define what is acceptable and what is normal for any particular screen; for example, we may accept a cell based assay with borderline acceptance criteria and an average robust Z′-factor of 0.4, and we may set performance expectations that we will fail plates in screening if their robust Z′-factor is less than 0.3. We often set greater expectations for biochemical assays where we commonly see an average robust Z′-factor of 0.7 and will fail plates with robust Z′-factors of less than 0.5. This may seem like a double standard. However, the data we have generated tell us the normal behaviours of an assay and we commit to screening once we have assessed this. Additionally, where a screening plate deviates from the normal behaviour this gives us reason to suspect that this plate is different and should not be included in the analysis.
As we move towards using rarer cell types and more complex biological read outs we will need different approaches such as screening smaller numbers of compounds using either replicates in single shot or dose response screening. As technologies progress and we start to look at single cells, our understanding of what a robust assay is and how to define such assays will have to evolve markedly as will the methods used to validate such screens. It is our belief, however, that similar criteria and advice will result in the continued use of HTS libraries and screening processes such that robust assays and valuable data can be generated to progress drug discovery projects.
References
- R. Macarron , M. N. Banks , D. Bojanic , D. J. Burns , D. A. Cirovic , T. Garyantes , D. V. Green , R. P. Hertzberg , W. P. Janzen , J. W. Paslay , U. Schopfer and G. S. Sittampalam , Nat. Rev. Drug Discovery, 2011, 10 , 188 CrossRefCASPubMed .
- D. C. SwinneyJ. Biomol. Screening, 2013, 18 , 1186 CrossRefPubMed .
- J. Zhang , T. Chung and K. Oldenburg , J. Biomol. Screening, 1999, 4 , 67 CrossRefPubMed .
- J. Baell and M. A. Walters , Nature, 2014, 513 , 481 CrossRefCASPubMed .
- Genedata Screener, www.genedata.com (accessed March 2016).
- N. Malo , J. A. Hanley , S. Cerquozzi , J. Pelletier and R. Nadon , Nat. Biotechnol., 2006, 24 , 167 CrossRefCASPubMed .
- C. Brideau , B. Gunter , B. Pikounis and A. Liaw , J. Biomol. Screening, 2003, 8 , 634 CrossRefPubMed .
- Z. Wu , D. Liu and Y. Sui , J Biomol. Screening, 2008, 13 , 159 CrossRefCASPubMed .
- C. Murie , C. Barette , J. Button , L. Lafanechere and R. Nadon , J. Biomol. Screening, 2015, 20 , 230 CrossRefPubMed .
- V. Makarenkov , P. Zentilli , D. A. Kevorkov , D. A. Gagarin , N. Malo and R. Nadon , Bioinformatics, 2007, 23 , 1648 CrossRefCASPubMed .
- V. Makarenkov , D. Kevorkov , P. Zentilli , A. Gagarin , N. Malo and R. Nadon , Bioinformatics, 2006, 22 , 1408 CrossRefCASPubMed .
- M. J. Wigglesworth , D. C. Murray , C. J. Blackett , M. Kossenjans and J. W. M. Nissink , Curr. Opin. Chem. Biol., 2015, 26 , 104 CrossRefCASPubMed .
- J. M. Bland and D. G. Altman , Lancet, 1986, 327 , 307 CrossRef .
© The Royal Society of Chemistry 2017 (2016)
Jpg"ppnbsp;ppnbsp;ppnbsp;ppnbsp;ppnbsp;ppnbsp;ppnbsp;ppnbsp;ppnbsp;ppnbsp;ppnbsp;ppnbsp;ppnbsp;ppnbsp;ppnbsp;ppnbsp;ppnbsp;ppnbsp;ppnbsp;ppnbsp;ppnbsp;ppnbsp;ppnbsp;ppnbsp;ppnbsp;ppnbsp;ppnbsp;ppnbsp;ppnbsp;ppnbsp;ppnbsp;ppnbsp;ppnbsp;ppnbsp;ppnbsp;ppnbsp;ppnbsp;ppnbsp;ppnbsp;ppnbsp;ppnbsp;ppnbsp;ppnbsp;ppnbsp;ppnbsp;ppnbsp;ppnbsp;ppnbsp;ppInternet Download Manager IDM 6. 15 Build 15 RARppnbsp;ppWhat,,Is,,the,,FileHippo,,Safety,,Guarantee?,,We,,know,,how,,important,,it,,is,,to,,stay,,safe,,online,,so,,FileHippo,,is,,using,,virus,,scanning,,technology,,provided,,by,,Avira,,to,,help,,ensure,,that,,all,,downloads,,on,,FileHippo,,are,,safe.
,,Its,features,are,intelligent,dynamic,file,segmentation,and,safe,multipart,downloading,technology,to,accelerate,your,downloads. ,Full,,Programlar,,indir,,AntiVirs,,Gvenlik,,Programlar,,Genel,,eit,,Programlar,,Dini,,Programlar,,Dini,,Paylamlar,,Bakm,,Onarm,,Programlar,,Grafik,,ve,,Resim,,Programlar,,Office,,2003,,2007,,2010,,2013,,2016,,Full,,Eitim,,Setleri,,Hepsi,,1,,Arada,,AIO,,Programlar,,E-Book,,Kitap,,ndir,,Full,,nternet,,Programlar,,ndir,,Katlmsz,,Program,,ndir,,Portable,,Program,,ndir,,PC,,Driver,,Ses,,ve,,Video,,Programlar,,Mac,,OS,,X,,Programlar,,Linux,,Programlar,,ndir,,Gncel,,Serial,,Key,,Blm,,cretsiz,,Program,,ndir,,cretsiz,,Yasal,,Lisans,,!,,Masast,,Ekrankoruyucu,,Pc,,Tema,,ndir,,Wallpapers,,HD,,Resim,,indir,,Gncel,,letim,,sistemleri,,Gncel,,letim,,sistemleri,,ndir,,Lisanlama,,amp;,,Crack,,Windows,,7,,Srmler,,Windows,,10,,Windows,,8,,Windows,,8.
.What’s New in the Lab DJ 1.2.5 serial key or number?
Screen Shot
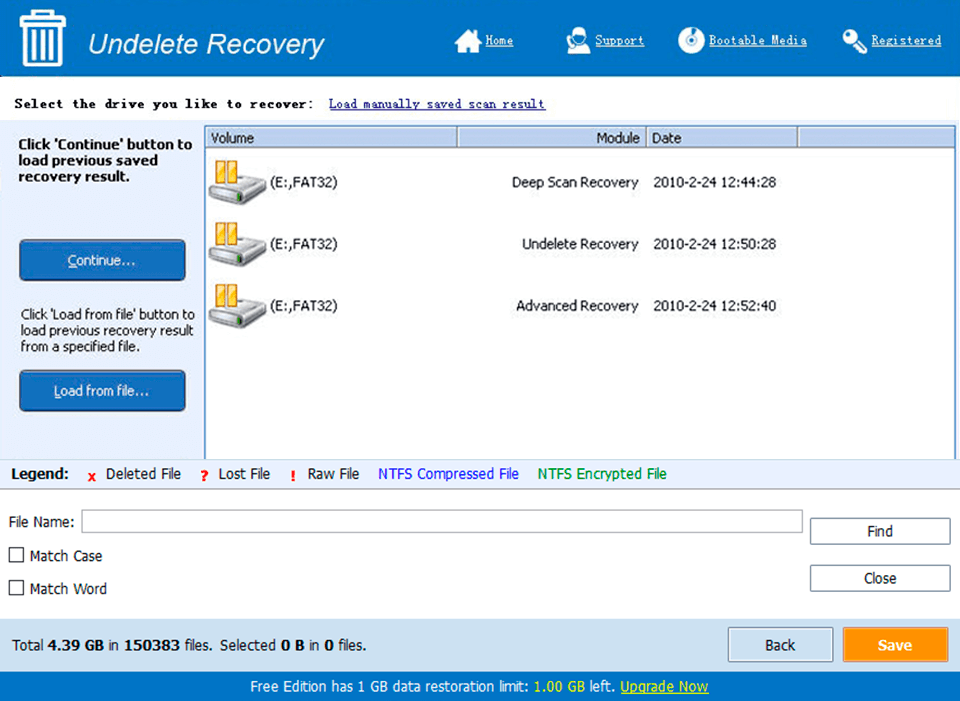
System Requirements for Lab DJ 1.2.5 serial key or number
- First, download the Lab DJ 1.2.5 serial key or number
-
You can download its setup from given links: