
BCL keygen

BCL keygen
miR-155 mediates arsenic trioxide resistance by activating Nrf2 and suppressing apoptosis in lung cancer cells
Abstract
Arsenic trioxide (ATO) resistance is a challenging problem in chemotherapy. However, the underlying mechanisms remain to be elucidated. In this study, we identified a high level of expression of miR-155 in a human lung adenocarcinoma A549R cell line that is highly resistant to ATO. We showed that the high level of miR-155 was associated with increased levels of cell survival, colony formation, cell migration and decreased cellular apoptosis, and this was mediated by high levels of Nrf2, NAD(P)H quinone oxidoreductase 1 (NQO1), heme oxygenase-1 (HO-1) and a high ratio of Bcl-2/Bax. Overexpression of the miR-155 mimic in A549R cells resulted in increased levels of colony formation and cell migration as well as reduced apoptosis along with increased Nrf2, NQO1 and HO-1. In contrast, silencing of miR-155 expression with its inhibitor in the cells, significantly decreased the cellular levels of Nrf2, NQO1 and HO-1 as well as the ratio of Bcl-2/Bax. This subsequently reduced the level of colony formation and cell migration facilitating ATO-induced apoptosis. Our results indicate that miR-155 mediated ATO resistance by upregulating the Nrf2 signaling pathway, but downregulating cellular apoptosis in lung cancer cells. Our study provides new insights into miR-155-mediated ATO resistance in lung cancer cells.
Introduction
Arsenic trioxide (As2O3, ATO) has been successfully used in the treatment of relapsed/refractory acute promyelocytic leukemia (APL) since 1970s1. It is also used as a treatment of solid tumors such as hepatic sarcoma, prostate, and renal cancer among others2,3,4. It has been shown that ATO can induce cancer cell death by causing oxidative stress, DNA damage, and apoptosis5. Studies from our group and others have demonstrated that ATO also causes cell death in lung cancer cells6,7 indicating that ATO may be employed for lung cancer treatment. However, the doses for ATO to induce lung cancer cell death are much higher than those for the treatment of hematologic malignancies6,7,8, indicating that lung cancer cells are more resistant to ATO than hematologic cancer cells. Since a high dose of ATO can result in severe side effects9, this hinders the preclinical trials of ATO for lung cancer treatment. Thus, it is critically important to study the mechanisms underlying ATO resistance of lung cancer cells as this will help identify novel targets for attenuating ATO resistance, thereby facilitating the application of ATO as a new treatment for lung cancer.
One of the important mechanisms that underlie anticancer drug resistance is the high level and capacity of antioxidants in cancer cells10, which are primarily regulated by the nuclear factor (erythroid-derived 2)-like 2 (Nrf2) and kelch-like ECH-associated protein-1 (KEAP1) signaling pathway, one of the most important cell defense and survival pathways11. Nrf2 is a critical transcription regulator of a series of antioxidants and detoxification enzymes. By uncoupling with KEAP1, Nrf2 initiates the expression of antioxidant genes including NAD(P)H quinone oxidoreductase 1 (NQO1) and heme oxygenase-1 (HO-1)11,12. However, previous studies have shown that cancer cells that exhibit a high level of Nrf2 are less sensitive to chemotherapeutic agents13. Moreover, an aberrant accumulation of Nrf2 in cancer cells confers cancer resistance to chemotherapeutic agents13. Because this can create an environment that promotes cancer cell growth and metastasis, but prevents cancer cells from apoptosis, thereby leading to tumor reoccurrence and poor prognosis in cancer patients12. Our previous studies have shown that ATO significantly increases the level of Nrf2 in a human lung carcinoma cell line, A549 cell line14, suggesting that upregulation of Nrf2 is involved in resistance of A549 cells to ATO. However, the mechanism underlying Nrf2-mediated cellular resistance to ATO in lung cancer cells remains to be elucidated.
MicroRNAs (miRNAs) are a class of small non-coding RNAs (19-25 nt) that regulate protein translation and stability of mRNA15. miRNAs downregulate gene expression by binding to the 3′-untranslated region (3′-UTR) of a target mRNA, thereby inducing degradation of mRNAs and silencing the expression of a target gene15. It has been found that miRNAs play critical roles in many biological processes including cell proliferation and survival15. Dysregulation of miRNAs modulates the initiation and progression of cancer16. Moreover, a growing body of evidence indicates that several miRNAs may mediate cellular resistance to chemotherapy and radiotherapy in various types of tumors and cancer, in particular, lung cancer17. Among all of the identified miRNAs, miR-155 is the one that has been characterized extensively. miR-155 is generated from an exon of a non-coding RNA known as B-cell Integration Cluster (BIC)18. It is involved in cancer initiation and progression as well as the development of cellular resistance to chemotherapeutic agents17,19,20,21. A previous study has shown that the level of miR-155 in lung cancer tissue is much higher than that in normal tissue22. Moreover, lung adenocarcinoma patients who exhibited a high level of miR-155 in the cancer tissue usually had poor prognosis20,22. Inhibition of miR-155 expression suppressed cancer cell proliferation and promoted apoptosis, thereby sensitizing cancer cells to chemotherapeutic agents, cisplatin and doxorubicin19,21. Interestingly, it has been also shown that miR-155 can upregulate NQO1 and HO-1 through activation of the Nrf2 signaling pathway, thereby protecting cells against oxidative stress23,24. This further indicates that miR-155 can modulate cell proliferation and apoptosis via regulation of cell redox homeostasis. Our previous results have shown that a high dose of ATO can reduce the total antioxidant capacity of lung cancer cell (A549) by inhibiting cellular expression of miR-155, leading to cancer cell death (unpublished data). However, the mechanisms by which A549 cells develop ATO resistance through miR-155 remains unknown. To address this, we established a lung cancer cell line that is highly resistant to ATO, the A549R cell line, as a model to study the mechanisms by which miR-155 mediates ATO resistance in lung cancer cells. We discovered that a high level of miR-155 and activation of the Nrf2 signaling pathway along with decreased cellular apoptosis led to ATO resistance of A549R. This was further confirmed by the fact that overexpression of the miR-155 mimic enhanced ATO resistance of A549 via increased levels of NQO1 and HO-1 as well as an increased ratio of Bcl-2/Bax, whereas silencing of miR-155 with its inhibitor significantly increased the cellular sensitivity to ATO, and this resulted from decreased levels of NQO1 and HO-1 proteins as well as an decreased ratio of Bcl-2/Bax. The results indicated that miR-155 mediated cellular ATO resistance by activating the Nrf2 signaling pathway and suppressing apoptosis. Our study provided new insights into the mechanisms underlying ATO resistance in lung cancer cells. We suggest that miR-155 can be developed as a new therapeutic target for combating ATO resistance in lung cancer.
Results
The ATO resistance of A549R cells
To determine if A549R cells exhibit a high level of resistance to ATO, we initially measured the viability of A549R cells under treatment of different doses of ATO in comparison to that of A549 cells with MTT assay. We found that the viability of A549 cells was significantly decreased by ATO that ranged from 2.5 µM to 30 µM (Fig. 1A). Treatment with 10 µM ATO for 72 hours, led to the death of more than 50% of A549 cells, whereas treatment with 20 µM–30 µM ATO resulted in the death of 80% of A549 cells (Fig. 1A). In contrast, under the same conditions, 10 µM ATO did not exhibit any cytotoxic effects on A549R cells, and 20 µM ATO only led to 20% cell death (Fig. 1A). The viability of A549R cells decreased along with increasing concentrations of ATO above 20 µM. The concentrations of ATO that caused the death of half of A549R cells fell into the range of 60 µM–80 µM, which were about 10-fold higher than that of A549 cells. The 50% inhibitory concentrations (IC50) of ATO for A549 and A549R cells were 8.02 µM and 76.81 µM, respectively. Based on the IC50, the drug resistance index of A549R cells was calculated as 9.58. The results indicated that A549R cells exhibited a significantly high level of resistance to ATO. To further verify the high level of resistance of A549R cells to ATO, we determined the colony formation of A549R and A549 cells without or with treatment of 20 µM ATO for 24 hours (Fig. 1B and C). Without treatment of ATO, both A549 and A549R cells exhibited a similar percentage of colony formation with 25.80% from A549 cells and 27.83% from A549R cells, respectively (Fig. 1B and C). With the treatment of 20 µM ATO, A549 cells exhibited only 3.33% of colony formation, whereas A549R cells exhibited 24.37% of colony formation (P < 0.05) (Fig. 1B and C). The results confirmed that A549R cells exhibited much higher resistance to ATO than A549 cells. We then examined the migration of A549R cells under ATO treatment to verify the drug resistant phenotype with a two-chamber system composed of an upper and lower chamber. Cell migration was measured by counting the number of cells which migrated from the upper chamber to the lower chamber (Fig. 1D and E). As shown in Fig. 1D and E, without ATO treatment, a similar number of A549 (361) and A549R cells (430) migrated from the upper chamber into lower chamber indicating that they have the similar ability of migration and survival. However, under treatment of 20 µM ATO, only 33 A549 cells migrated, whereas 421 A549R cells migrated. This indicates that A549R cells readily survived to migrate under the ATO treatment (Fig. 1E and F). Thus, the results demonstrated that A549R cells were much more resistant to ATO than A549 cells.
A549R cells exhibit poor apoptotic activity under ATO treatment
Since apoptosis can mediate ATO-induced cell death, we asked whether the high level of resistance of A549R cells to ATO was due to the modulation of apoptotic process. To address this question, we determined the percentage of apoptotic cells in untreated A549 and A549R cells as well as in the cells treated with 20 µM ATO for 24 hours and the level of apoptotic proteins in the cells. We found that lower than 0.36% of apoptotic cells were detected in untreated A549 and A549R cells (Fig. 2A, left panels and Fig. 2B). However, 20 μM ATO increased the percentage of apoptotic cells in A549 and A549R cells to 18% and 3%, respectively (Fig. 2A, right panels and Fig. 2B). Thus, A549R cells exhibited 6-fold lower percentage of apoptotic cells than A549 cells (Fig. 2A and B). This indicated that A549R cells exhibited much lower apoptotic activity than A549 cells under treatment of ATO. To further identify the molecular basis underlying the phenomena, we determined the expression levels of the pro-apoptotic protein Bax and anti-apoptotic protein Bcl-2 in the cells. We found that the level of Bax in A549R cells was lower than that in A549 cells (Fig. 2C and D), whereas the level of Bcl-2 in A549R cells was higher than that in A549 cells (Fig. 2C and D). This resulted in a lower ratio of Bax/Bcl-2 in A549R cells.
Nrf2 signaling pathway contributes to ATO resistance of A549R cells
Nrf2 mainly upregulates the expression of cellular antioxidant genes such as NQO1 and HO-112,13. Since our previous studies have shown that activation of Nrf2 is involved in ATO resistance in A549 cells14, it is conceivable that the Nrf2 signaling pathway may also mediate the high level of ATO resistance in A549R cells by increasing the cellular antioxidant capacity of the cells. To test this, we examined the distribution of Nrf2 in the nucleus and cytoplasm with immunofluorescence. We found that Nrf2 existed in both the nucleus and cytoplasm of A549 cells and A549R cells (Fig. 3A). However, the level of Nrf2 protein in the nucleus and cytoplasm of A549R cells was significantly higher than that in A549 cells (Fig. 3B and C). Accordingly, the levels of antioxidant proteins, HO-1 and NQO1 in A549R cells were also much higher than those in A549 cells (Fig. 3D and E). The results indicate that the Nrf2 signaling pathway was more active in A549R cells than in A549 cells.
miR-155 promotes the resistance of A549R cells to ATO
miR-155 expression is associated with increased cell proliferation and chemotherapeutic drug resistance such as cellular resistance to cisplatin and doxorubicin19,21. Recent studies have also shown that miR-155 can upregulate the expression of NQO1 and HO-1 gene to increase cellular antioxidative capacity and cell survival23,24. Thus, it is possible that miR-155 may mediate ATO resistance in A549R cells by upregulating NQO1 and HO-1 gene expression. To test this, we measured the level of miR-155 in A549 and A549R cells and found that the level of miR-155 in A549R cells was significantly higher than that in A549 cells (Fig. 4A). Moreover, introduction of the miRNA-155 mimic (M) or the miRNA-155 inhibitor (I) into A549R cells increased or decreased the level of miR-155 by about 2-fold compared with that of the miR-155 mimic control (MC) or the miR-155 inhibitor control (IC) (Fig. 4A). The results indicate that the miR-155 mimic or the miR-155 inhibitor can be employed to effectively regulate the level of miR-155 in A549R cells. Further characterization of the effects of miR-155 on the growth and proliferation of A549R cells under ATO treatment showed that A549R cells transfected with the miR-155 mimic exhibited a higher percentage of colony formation (29.27%) than the control (24.43%) (P < 0.05) (Fig. 4B and C), whereas A549R cells transfected with the miR-155 inhibitor exhibited a lower percentage of colony formation (15.83%) than the control (24.50%) (P < 0.05) (Fig. 4B and C). Similarly, under treatment of 20 µM ATO, 509 A549R cells transfected with the miR-155 mimic, migrated, whereas 375 A549R cells transfected with the control vector, migrated indicating that upregulation of miR-155 promoted the migration and survival of A549R cells (Fig. 4D and E). However, under the same treatment, only a small number of A549R cells transfected with the miR-155 inhibitor, migrated (Fig. 4D and E) indicating that downregulation of miR-155 suppressed cell migration and survival. The results indicate that overexpression of miR-155 promoted A549R cell survival and cellular resistance to ATO, whereas suppression of miR-155 expression inhibited A549R survival and reduced cellular resistance to ATO. This further indicated that miR-155 mediated ATO resistance of A549R cells.
miR-155 downregulates ATO-induced apoptosis in A549R cells
Since we found that ATO-induced apoptosis was significantly reduced in A549R cells (Fig. 3), it is possible that apoptosis in A549R cells may be downregulated by miR-155. To test this possibility, we determined the percentage of apoptotic cells in A549R cells that were transfected with the miR-155 mimic or the miR-155 inhibitor under treatment of 20 µM ATO for 24 hours (Fig. 5A and B). The results showed that ATO resulted in a low percentage of apoptotic cells (0.8%) in A549R cells transfected by the miR-155 mimic, and a high percentage of apoptotic cells (12.9%) in the cells transfected with the miR-155 inhibitor (Fig. 5A and B, right panels). However, ATO treatment only resulted in 3.27% and 3.13% apoptotic cells in A549R cells transfected with the vector controls of the miR-155 mimic and the miR-155 inhibitor, respectively (Fig. 5A and B, left panels). This indicated that miR-155 efficiently downregulated apoptosis. Since a low ratio of Bax/Bcl-2 can mediate anti-cancer drug resistance by preventing the initiation of apoptosis25, we then asked if up- or downregulation of miR-155 could up- or downregulate apoptosis by modulating the ratio of Bax/Bcl-2. To address this, we examined the expression level of Bax and Bcl-2 proteins in A549R cells transfected with the miR-155 mimic or the miR-155 inhibitor. We found that in A549R cells transfected with the miR-155 mimic, the level of Bcl-2 and Bax was 1.46- and 0.64-fold of those in untransfected cells, respectively (Fig. 5C and D). However, the levels of Bcl-2 and Bax in A549 cells transfected with the miR-155 inhibitor was 0.71- and 1.83-fold of those in untransfected cells (Fig. 5E and F). Thus, the miR-155 mimic decreased the ratio of Bax/Bcl-2 in A549R cells to 0.44, whereas the miR-155 inhibitor elevated the Bax/Bcl-2 ratio to 2.58. The results indicate that miR-155 suppressed the initial response of A549R cells to apoptosis by decreasing the ratio of Bax/Bcl-2 through upregulating Bcl-2 and downregulating Bax.
Effects of miR-155 on the expression of Nrf2, HO-1 and NQO1 in A549R cells
Previous studies have shown that the expression of cellular antioxidants, HO-1 and NQO1can be regulated by miR-15523,24. We have also discovered that HO-1 and NQO1 are involved in cellular ATO drug resistance26. To further determine if miR-155 can mediate the resistance of A549R cells to ATO by regulating cellular levels of Nrf2, HO-1 and NQO1, we examined the levels of Nrf2, HO-1 and NQO1 in A549R cells transfected with the miR-155 mimic, the miR-155 inhibitor, the miR-155 mimic control and miR-155 inhibitor vector control, respectively. We found that the levels of Nrf2, HO-1 and NQO1 proteins were increased by about 1.5-fold in A549R cells transfected with the miR-155 mimic compared with those in the cells transfected with the mimic vector control and untransfected A549R cells (P < 0.05) (Fig. 6A and B). In contrast, the levels of HO-1 and NQO1 proteins were decreased by about 0.6-fold in A549R cells transfected with the miR-155 inhibitor compared with those in cells transfected with the inhibitor vector control (Fig. 6C and D). The results indicate that miR-155 activated the Nrf2 signaling pathway to increase the levels and capacity of antioxidants in A549R cells. This in turn enhanced the resistance of A549R cells to ATO.
Discussions
In this study, we initially characterized a lung adenocarcinoma cell line, i.e. A549R cells that exhibited a significantly high level of resistance to ATO than A549 cells (Figs 1 and 2). We then explored the mechanisms underlying the ATO-resistance of A549R cells. We provided the first evidence that miR-155 mediated a high level of ATO-resistance in a lung cancer cell line, A549R cells by suppressing cellular apoptotic activities and upregulating the expression of antioxidants NQO1 and HO-1 via activation of the Nrf2 signaling pathway (Figs 2–6), and this subsequently protected A549R cells from ATO-induced apoptotic cell death, thereby promoting cell survival (Figs 4 and 5). The results support a model that miR-155 mediates the resistance of A549R cells to ATO through a pathway in which miR-155 increases the level of Nrf2 in the nucleus and cytoplasm of A549R cells. This subsequently upregulates the expression of antioxidant proteins NQO1 and HO-1 that protect cells from ATO-induced oxidative stress. On the other hand, miR-155 upregulates Bcl-2 and downregulates Bax, resulting in a high ratio of Bcl-2/Bax that subsequently prevents apoptosis. All of these promote cell survival and a high level of cellular resistance to ATO (Fig. 7).
miR-155 is implicated in the initiation and progression of cancer as well as the development of drug resistance in cancer cells22,23. It has been shown that silencing of miR-155 leads to overexpression of the pro-apoptotic protein, Apaf-1, which increases the sensitivity of A549 cells to cisplatin21. Similarly, suppression of miR-155 in doxorubicin-resistant A549 cells (A549/dox) can significantly reduce doxorubicin resistance19. The results indicate that miR-155 plays a universal role in mediating anticancer drug resistance in A549 cells. In this study, we further demonstrated that miR-155 was also involved in mediating the resistance of A549R cells to ATO. Since miR-155 can upregulate NQO1 and HO-1 through activation of the Nrf2 signaling pathway to promote cellular redox homeostasis and cell survival23,24, it appears that miR-155 may also mediate the resistance of A549R lung cancer cells to ATO by activating the Nrf2 signaling pathway and its downstream target genes. Indeed, this notion was supported by our results showing that the expression of Nrf2, NQO1 and HO-1 was upregulated along with upregulation of miR-155 in A549R cells (Figs 3 and 4A). This was further supported by the fact that overexpression of miR-155 in A549R cells increased the levels of Nrf2, NQO1 and HO-1, whereas suppression of the expression of miR-155 decreased the levels of the antioxidant proteins (Fig. 6). Thus, our results indicate that miR-155 mediated ATO resistance in A549R cells through the same cell signaling pathways as it mediates cellular resistance to other chemotherapeutic drugs. Our study further suggests that miR-155 plays a central role in mediating anticancer resistance through regulating the Nrf2 signaling pathway and can be developed as a new target for combating drug resistance of lung cancer.
Our results are consistent with previous reports showing that miR-155 plays an important role in anticancer drug resistance in cancer cells19,21. However, a recent study has shown that miR-155 can suppress the Nrf2 signaling pathway in responding to oxidative stress induced by a different chemical compound in a different type of cells. This was demonstrated by a role of miR-155 in mediating hepatocytotoxicity induced by an environmental toxicant, perfluorooctane sulfonate (PFOS) in vitro and in vivo27. In contrast to its role in mediating ATO resistance in lung cancer cells, miR-155 significantly suppresses Nrf2 expression and activation in normal hepatocytes of rats in responding to PFOS-induced oxidative stress
Oxidative stress mediates the apoptosis and epigenetic modification of the Bcl-2 promoter via DNMT1 in a cigarette smoke-induced emphysema model
- Huihui Zeng1,2,3,
- Tiao Li1,2,3,
- Xue He1,2,3,
- Shan Cai1,2,3,
- Hong Luo1,2,3,
- Ping Chen1,2,3 &
- Yan ChenORCID: orcid.org/0000-0002-7713-69131,2,3
Respiratory Researchvolume 21, Article number: 229 (2020) Cite this article
321 Accesses
2 Altmetric
Metrics details
Abstract
Background
Emphysema is a crucial pathological characteristic of chronic obstructive pulmonary disease (COPD). Oxidative stress, apoptosis and epigenetic mechanisms contribute to the pathogenesis of emphysema. However, an attempt to accurately identify whether these mechanisms interact with each other and how they are triggered has never been conducted.
Method
The total reactive oxygen species (ROS) level, pulmonary apoptosis and B-cell lymphoma/leukemia-2 (Bcl-2) expression, an apoptosis regulator, were detected in samples from COPD patients. Bisulfite sequencing PCR (BSP) was conducted to observe the alterations in the methylation of the Bcl-2 promoter in specimens. The dysregulation of DNA methyltransferase enzyme 1 (DNMT1), a vital DNA methyltransferase enzyme, in the lungs of patients was confirmed through western blotting. To find out interactions between oxidative stress and DNA methylation in emphysema, mouse models were built with antioxidant treatment and DNMT1 silencing, and were examined with the pulmonary apoptosis, Bcl-2 and DNMT1 levels, and epigenetic alterations of Bcl-2.
Results
Higher ROS levels and pulmonary apoptosis were observed in COPD patients than in healthy controls. Downregulated Bcl-2 expression with increased promoter methylation and DNMT1 protein expression was found in COPD patients. Antioxidant treatment reduced the level of ROS, DNMT1 protein and emphysematous progression in the smoking models. Following DNMT1 blockade, smoking models showed improved lung function, pulmonary apoptosis, emphysematous progression, and increased Bcl-2 protein level with less promoter methylation than emphysema mice.
Conclusion
Cigarette-induced oxidative stress mediates pulmonary apoptosis and hypermethylation of the Bcl-2 promoter in emphysema models through DNMT1.
Introduction
Chronic obstructive pulmonary disease (COPD) is a worldwide public health burden because of its high prevalence [1]. A recent study [2] showed that the estimated total number of individuals aged 20 years or older with spirometry-defined COPD in China was 99.9 million in 2015. Cigarette smoking is an established risk factor for COPD, and smoking cessation seems to be the most effective intervention for COPD [1,2,3]. However, an abundance of studies have demonstrated that pulmonary pathological progress was ongoing and could not be reversed in COPD patients after smoking cessation [4,5,6]. There are several mechanisms that contribute to the pathogenesis of emphysema, a prominent pathological hallmark of COPD, such as persistent inflammation, proteolytic/anti-proteolytic imbalance, oxidative stress, and apoptosis [4, 7, 8].
Several studies [9,10,11,12] have demonstrated that oxidative stress was a critical factor, that links inflammation, excessive matrix proteolysis and direct damage to DNA in emphysema. Our previous study and some others [13,14,15,16] showed that oxidative stress played an important role in apoptosis in COPD patients and models. Apoptosis is a highly regulated cell suicide program that can be regulated by B-cell lymphoma/leukemia-2 (Bcl-2) family proteins [17, 18]. We also demonstrated that Bcl-2 protein level was decreased in emphysema models and COPD patients, suggesting that Bcl-2 is involved in COPD pathogenesis [19]. The epigenetic regulation of DNA can stably propagate during a lifetime and might account for ongoing disease progression [20, 21]. Promoter methylation is an emerging and important epigenetic regulatory mechanism, that inhibits target gene transcription and blocks gene expression [22, 23]. Our previous studies [19, 24] found a high methylation status of the whole genome and hypermethylation of the Bcl-2 promoter in COPD patients and emphysema models, and demethylation treatment could protect models from emphysema. DNA methyltransferase 1 (DNMT1) is regarded as the primary methyltransferase, contributing to de novo DNA methylation and maintenance [25]. Based on the above studies, we postulated that cigarette smoke (CS)-induced oxidative stress mediates apoptosis and Bcl-2 methylation via DNMT1 in emphysema.
Results
Demographic, pulmonary function and morphological findings in COPD patients
We have collected 27patients, who were underwent the excision of peripheral solitary pulmonary nodule or pulmonary lesion. Nine patients are normal nonsmokers, and 9 cases were non-COPD smokers. The last 9 cases were smokers with spirometry-defined COPD. There was no significant difference in the gender ration or age among the three groups (Table 1). The smoking history of non-COPD smokers and COPD smokers was analyzed, and no difference in smoking history was found between the two groups (P = 0.23, Table 1).
Compared with the other two groups, the COPD smokers showed destroyed pulmonary architecture and function (P < 0.05 by one-way ANOVA and least significant difference (LSD) test, Table 1 and Fig. 1a). Histologically, the nonsmokers presented a well-fixed normal pulmonary parenchyma with normal airways (Fig. 1a). Non-COPD smokers did not exhibit emphysematous changes, but presented a thicker alveolar septum than nonsmokers (mean alveolar septal thickness, MAST; Fig. 1b). COPD patients showed significant emphysematous disorder with an increased mean linear intercept (MLI) and destructive index (DI; Fig. 1c, d).
Aggravated pulmonary apoptosis and oxidative stress in the lungs of COPD patients
Compared to that in the lungs of nonsmokers and smokers without COPD, the apoptosis index (AI) was increased in the lungs of COPD patients (Fig. 2a, b). Interestingly, there was no difference in the pulmonary AI (%) between the nonsmokers and smokers without COPD (3.73 ± 1.57 vs. 3.99 ± 1.75, P < 0.01 by the Kruskal-Wallis test, Fig. 2a, b). This finding suggests that CS is not the only reason for apoptosis in COPD, and there might be mechanisms beyond direct CS damage. The level of reactive oxygen species (ROS) in tissues from COPD patients was higher than that in tissues from nonsmokers (Fig. 2c), indicating that there was a higher oxidant burden in COPD. In contrast, the smokers without COPD did not show a significant change in ROS levels (Fig. 2c).
Dysregulation of Bcl-2 and DNMT1 protein levels in lungs from COPD patients
Lung tissues from nonsmokers, non-COPD smokers and COPD patients were analyzed for the expression of Bcl-2, a well-known apoptosis regulator, by western blotting. Bcl-2 protein in lung tissue from COPD patients was lower than that in lung tissue from nonsmokers (Fig. 3a, b). To discover whether DNA methylation is involved in COPD, DNMT1 protein, the DNA methyltransferase, was analyzed in lung tissues (Fig. 3c). Immunoblotting showed that DNMT1 expression in lung tissue from non-COPD smokers and COPD patients was higher than that in lung tissue from nonsmokers (0.44 ± 0.12 and 0.73 ± 0.06 vs. 0.29 ± 0.11, P < 0.01 by one-way ANOVA and LSD test, Fig. 3c, d). In addition, the COPD group presented higher DNMT1 expression than the non-COPD smoker group (P < 0.01 by one-way ANOVA and LSD test, Fig. 3c, d).
Hypermethylation of the Bcl-2 promoter in COPD patients
Given the higher expression of DNMT1 and lower expression of Bcl-2 in COPD patients than in healthy controls, we conducted bisulfite sequencing PCR (BSP) to detect the methylation status of the Bcl-2 promoter. The sequence results demonstrated that the COPD patients had an elevated level of Bcl-2 promoter methylation (Fig. 4). As the results of Bcl-2 protein detection, BSP showed that there was no significant difference in Bcl-2 methylation levels between the nonsmoker and non-COPD smoker groups (P = 0.756, Fig. 4). Considering the simultaneously increased DNMT1 expression and methylation level, it is possible to assume that the upregulated DNMT1 level leads to the hypermethylation of the Bcl-2 promoter in COPD patients.
CS-induced oxidative stress participates in emphysema, pulmonary apoptosis and lung function damage
To confirm that oxidative stress contributes to COPD pathogenesis, we treated mice with CS and vitamin E (Vit E), one of the most common antioxidant reagents. Consistent with previous studies [13,14,15], we found that CS exposure caused increased ROS levels in lung tissue (Fig. 5a). Antioxidant feeding with Vit E prevented the increased ROS levels in CS exposed mice (Fig. 5a). Moreover, CS-treated mice exhibited emphysematous changes with aggravated MLI, DI and AI (Fig. 5b, c, d, and e). Coincidentally, the mice exposed to CS showed impaired pulmonary function, including deteriorated tidal volume (TV, mL), dynamic compliance (Cdyn, mL/cm H2O) and airway resistance (RI, cm H2O/mL/min; Fig. 5f, g). Interestingly, antioxidant treatment also alleviated the pulmonary morphological and functional damage caused by CS (Fig. 5b, c, d, e, f, and g). This finding suggests that CS-induced oxidative stress plays a role in emphysema, pulmonary apoptosis and lung function damage through oxidative stress.
CS-induced oxidative stress is involved in the dysregulation of Bcl-2, cleaved caspase-3 and DNMT1 protein expression and DNA hypermethylation of the Bcl-2 promoter
Because of the increased apoptosis in COPD patients’ lungs, we detected the apoptosis regulator Bcl-2 and the apoptosis inducer caspase-3 in mouse models. Compared to the control mice, the CS exposed mice had lower Bcl-2 protein expression in the pulmonary tissue (0.62 ± 0.03 vs. 0.37 ± 0.05, P < 0.01 by one-way ANOVA and LSD test, Fig. 6a, b). Correspondingly, we also found that the level of cleaved caspase-3 protein in the CS group was higher than that in control group (0.75 ± 0.09 vs. 0.15 ± 0.05, P < 0.01 by the Kruskal-Wallis test, Fig. 6a, c). Given the hypermethylation of the Bcl-2 promoter in the lungs from COPD patients, we detected DNMT1 protein expression and the DNA methylation level of Bcl-2 in pulmonary tissue from mouse models. Immunoblotting showed that DNMT1 protein level was upregulated in the lungs from the CS group (Fig. 6a, d). BSP was subsequently performed, and showed that CS led to the hypermethylation of the Bcl-2 promoter (Fig. 6e). In contrast, the antioxidant reagent ameliorated the CS-induced dysregulation of the apoptosis-associated proteins Bcl-2 and cleaved caspase-3. Moreover, it prevented the alternation in DNMT1 protein level and the CS-induced epigenetic modification of Bcl-2 (Fig. 6a, b, c, d, and e). This finding indicates that CS-induced oxidative stress causes the dysregulation of apoptosis-associated proteins and gene methylation status, and ultimately initiates apoptosis.
Bcl-2 Proteins Regulate Mitophagy in Lipopolysaccharide-Induced Acute Lung Injury via PINK1/Parkin Signaling Pathway
Abstract
Mitophagy is involved in sepsis-induced acute lung injury (ALI). Bcl-2 family proteins play an important role in mitochondrial homeostasis. However, whether targeting Bcl-2 proteins (Bcl-2 and Bad) could influence mitophagy in ALI remains unclear. In this study, lipopolysaccharide (LPS) was used to induce injury in A549 cells and ALI in mice. LPS treatment resulted in elevated cell apoptosis, enhanced mitophagy, decreased Bcl-2 expression, increased Bad expression, and activation of PINK1/Parkin signaling in cells and lung tissues. Both Bcl-2 overexpression and Bad knockdown attenuated LPS-induced injury, inhibited cell apoptosis and mitophagy, and improved survival. Atg5 knockout (KO) inhibited LPS-induced cell apoptosis. Furthermore, Bcl-2 proteins regulated mitophagy by modulating the recruitment of Parkin from the cytoplasm to mitochondria via direct protein-protein interactions. These results were further confirmed in Park2 KO cells and Park2-/- mice. This is the first study to demonstrate that Bcl-2 proteins regulated mitophagy in LPS-induced ALI via modulating the PINK1/Parkin signaling pathway, promoting new insights into the mechanisms and investigation of therapeutic strategies for a septic patient with ALI.
1. Introduction
Acute lung injury (ALI) and acute respiratory distress syndrome (ARDS) contribute to mortality and morbidity in critically ill patients. ALI is a type of diffuse alveolar-capillary membrane injury characterized by pulmonary edema and atelectasis, and ARDS is a serious and life-threatening condition [1, 2]. Studies showed that inflammation, mitochondrial damage, and apoptosis are involved in the etiology of ALI/ARDS [3]. Despite recent progress, the mortality rate associated with ALI/ARDS patients remains high [4].
Mitophagy plays an important role in cell homeostasis by the degradation and removal of damaged mitochondria [5, 6]. However, excessive mitophagy may promote mitochondrial dysfunction and cause cell injury and death [7]. The phosphatase and tension homolog deleted on chromosome 10-induced kinase 1 (PINK1)/Parkin signaling pathway plays a key role in the process of mitophagy. PINK1 shuttles between the cytosol and mitochondria to interact with Parkin, a ubiquitin (Ub) E3 ligase encoded by the Park2 gene [8]. Under normal conditions, PINK1 is degraded by presenilin-associated rhomboid-like protease (PARL). During mitophagy with mitochondrial membrane potential dissipation, the activity of PARL is inhibited, promoting Parkin phosphorylation in the cytoplasm and recruitment to the mitochondria [9]. Polyubiquitinated proteins in the mitochondrial outer membrane trigger the migration of Ub and the LC3 binding adaptor protein p62. This molecule functions to link the damaged mitochondria and mitolysosomes [10, 11], which are formed by the fusion of mitophagosomes and lysosomes to mediate the degradation of the damaged mitochondria [12].
Bcl-2 has been well studied to exert antiapoptotic effects in various apoptotic cell and animal models [13, 14]. Bcl-2 may also regulate autophagy. Hollville et al. showed that Bcl-2 family proteins suppressed mitophagy by inhibiting Parkin translocation to depolarized mitochondria in Hela and HEK293T cells [15]. Bad, an upstream member of the Bcl-2 family, functions as a sensor of cell stress, damage, infection, growth factor deprivation, and apoptotic process [16]. Bad is a BH3-like protein that interacts with Bcl-2. The BH1-BH4 domain of Bcl-2 forms a hydrophobic groove structure and binds to Bad [17].
In this study, we evaluated the role of Bcl-2 proteins in the regulation of mitophagy in lipopolysaccharide- (LPS-) treated A549 cells and LPS-induced ALI in mice. We also investigated cell apoptosis and its relationship with mitophagy in LPS-induced ALI. The effects of Bcl-2 overexpression and Bad knockdown on mitophagy and apoptosis were assessed in vitro and in vivo. Furthermore, Park2 knockout (KO) cells, Agt5 KO cells, and Park2-/- mice were used to investigate the underlying mechanisms.
2. Results
2.1. LPS-Induced Injury in A549 Cells and ALI in Mice
CCK-8 and LDH assays showed significantly decreased cell viability and increased cell toxicity in A549 cells treated with 50 and 100 μg/ml LPS for 8 and 16 h (Figure 1(a)). We selected 50 μg/ml LPS exposure of 16 h for the following experiments. Next, we found LPS treatment led to elevated cell apoptosis (Figure 1(b)). The ratio of cleaved caspase3 to procaspase3 protein expression was significantly increased 8 and 16 h after LPS exposure (Figure 1(c)).
In mice, LPS induced inflammatory cell infiltration in the alveolar and connective tissue of the alveolar septum, with significant neutrophil aggregation around the pulmonary vessels and bronchi (Figure 1(d)). Lung tissues showed hyperemia, edema, alveolar diaphragm thickening, and early transparent membrane formation in the alveolar wall surface. In addition, increased inflammatory cells were found in the alveolar lavage fluid in LPS mice (Figure 1(d)). Lung injury scores were then calculated using the pathological ALI scoring system according to the American Thoracic Association criteria [18]. Compared with the control group, the LPS group showed significantly a higher lung injury score (Figure 1(e)), BALF protein content (Figure 1(f)), and wet/dry ratio (Figure 1(g)).
2.2. LPS-Induced Mitophagy in A549 Cells and Mouse Lung Tissues
In LPS-treated cells, we observed increased colocalization of LC3GFP and MitoTracker red (MTR) (Figure 2(a)). LPS resulted in deceased expression of mitochondrial outer membrane protein Tom20, mitochondrial inner membrane protein COX IV, and P62, with an increased LC3-II/LC3-I ratio (Figure 2(b)). Electron microscopic images showed increased mitophagy induced by LPS [19] (Figure 2(c)). We also found that LPS caused remarkable damage to the mitochondrial network and structure (Figure 2(d)). Under stress conditions, damaged mitochondria are isolated from the rest of the mitochondrial network or repaired via mitochondria fusion [20], which was regulated by a number of mitophagy-related proteins [21]. Mitofusin-2 (Mfn2) plays a critical role in mitochondrial fusion [22], while dynamin-related protein 1 (Drp1) promotes mitochondrial fission [21]. In this study, the protein expression of Mfn2 was reduced and Drp1 expression was increased by LPS (Figure 2(e)). In addition, LPS exposure significantly increased reactive oxygen species (ROS) levels (Figure 2(f)) and decreased ATP levels (Figure 2(g)) and resulted in decreased mitochondrial membrane potential (Figure 2(h)). These findings suggest that the activation of mitophagy plays a critical role in LPS-induced ALI.
Moreover, we used CRISPR-Cas9 gene editing technology to knock out the Atg5 gene, an important factor of mitophagy, in A549 cells (Figure S1A). Knockout of Atg5 has been indicated to inhibit mitophagy [23]. Our results showed that apoptosis induced by LPS was alleviated in Atg5 KO cells compared to wild-type cells (Figures S1B and S1C). Therefore, inhibition of mitophagy could attenuate apoptosis in LPS-induced ALI.
2.3. Bcl-2 Family Proteins and PINK1/Parkin Signaling during LPS Exposure
Western blot and RT-qPCR analyses showed that LPS exposure led to decreased Bcl-2 expression and increased expression of Bad and PINK1 at 4 and 8 h in A549 cells (Figures 3(a) and 3(b)). We also found downregulated Bcl-2 expression and upregulated Bad expression in LPS-induced ALI tissues (Figure 3(c)). By isolating the mitochondrial and cytoplasmic components of A549 cells, LPS-treated cells showed decreased Parkin expression in the cytoplasm and increased Parkin expression in the mitochondria (Figure 3(d)). In addition, we found increased expression and colocalization of Parkin and Tom20 during LPS treatment (Figure 3(e)). The colocalization of Parkin and Tom20 was evaluated by the calculation of Pearson’s correlation coefficient [24]. In line with our results, a previous study showed that Parkin is recruited from the cytoplasm to the dysfunctional mitochondria during mitochondrial depolarization [25]. Besides, the protein expression of other members of Bcl-2 family proteins including Bcl-xl, Bcl-w, and Bim was assessed. LPS treatment significantly decreased Bcl-xl and Bcl-w expression and increased Bim expression (Figure S2). These findings suggest the involvement of Bcl-2 family proteins and enhanced PINK1/Parkin-mediated mitophagy during LPS treatment.
2.4. Bcl-2 Overexpression Attenuated Cell Injury In Vitro and ALI In Vivo by Inhibiting Mitophagy
To explore the role of Bcl-2 in LPS-induced ALI, overexpression of Bcl-2 was carried out by transient transfection of A549 cells with the AAV-CMV-Bcl-2 vector (Figure 4(a)). Bcl-2 overexpression significantly increased cell viability (Figure 4(b)), restored total ATP (Figure 4(c)), and reduced ROS levels (Figure 4(d)) in LPS-treated cells. Reduced expression of cleaved caspase3 (Figure 4(e)) and Annexin V-FITC staining (Figure 4(f)) indicated that LPS-induced apoptosis was significantly alleviated by Bcl-2 overexpression. Bcl-2 overexpression also restored the expression of Tom20, COX IV, and mitofusin-2 (Mfn2) and inhibited dynamin-related protein 1 (Drp1) expression and LC3-II/LC3-I ratio (Figure 4(e)). Moreover, the reduced apoptosis was reversed by the use of mitophagy inducer antimycin A (500 nM) or CCCP (5 μM) (both from Selleck Chemicals, Houston, TX, USA) (Figure 4(f)). These findings suggest that Bcl-2 attenuates LPS-induced injury by inhibiting mitophagy. Bcl-2 family members have been shown to be associated with the remodeled mitochondrial networks [21]. JC-1 assays showed that Bcl-2 overexpression alleviated LPS-induced mitochondrial membrane potential dissipation, which was blocked by antimycin A or CCCP (Figure 4(g)).
The effects of Bcl-2 overexpression were also investigated in mice (Figure 4(h)). HE staining showed that Bcl-2 overexpression significantly reduced the inflammatory cell infiltration in lung tissues (Figure 4(i)) and improved lung injury scores (Figure 4(j)) during LPS-induced ALI. Furthermore, transmission electron microscopy showed fewer mitophagosomes in the Bcl-2 overexpression group compared to the control group (Figure 4(k)). Bcl-2 overexpression also reduced the wet/dry weight ratio in LPS-induced ALI tissues (Figure 4(l)). These findings suggest that LPS resulted in mitochondrial dysfunction, apoptosis, and excessive mitophagy, which was attenuated by Bcl-2 overexpression.
2.5. Bad Knockdown Attenuated Cell Injury In Vitro and ALI In Vivo by Inhibiting Mitophagy
To evaluate the role of Bad in LPS-induced ALI, Bad knockdown was achieved by transfection with shBad lentivirus (Figure 5(a)). Bad knockdown increased cell viability (Figure 5(b)) and total ATP (Figure 5(c)) and reduced ROS levels (Figure 5(d)). Bad knockdown also reduced the LC3-II/LC3-I ratio and the expression of cleaved caspase3 and Drp1 and restored the expression of Tom20, COX IV, and Mfn2 during LPS-induced cell injury (Figure 5(e)). In addition, Bad knockdown reduced the apoptosis rate (Figure 5(f)) and restored mitochondrial membrane potential during LPS treatment (Figure 5(g)).
What’s New in the BCL keygen?
Screen Shot
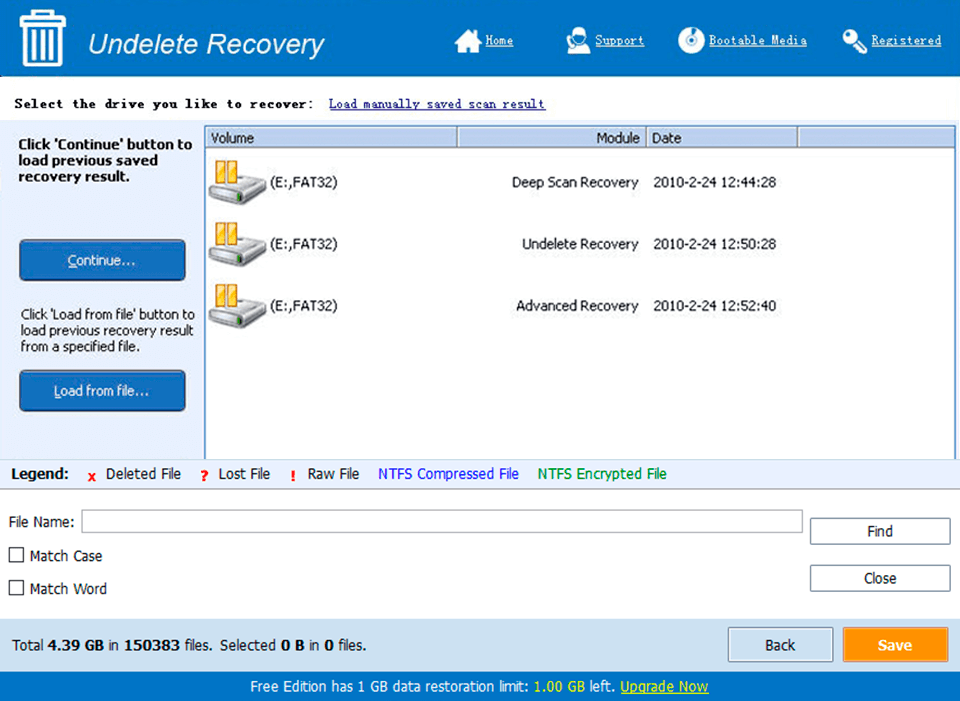
System Requirements for BCL keygen
- First, download the BCL keygen
-
You can download its setup from given links: