
Performance Diet Pro 2001 v3.0.6 serial key or number

Performance Diet Pro 2001 v3.0.6 serial key or number
Open Access
Peer-reviewed
- Per I. Rustad,
- Manuela Sailer ,
- Kristoffer T. Cumming ,
- Per B. Jeppesen ,
- Kristoffer J. Kolnes,
- Ove Sollie,
- Jesper Franch,
- John L. Ivy,
- Hannelore Daniel,
- Jørgen Jensen
- Per I. Rustad,
- Manuela Sailer,
- Kristoffer T. Cumming,
- Per B. Jeppesen,
- Kristoffer J. Kolnes,
- Ove Sollie,
- Jesper Franch,
- John L. Ivy,
- Hannelore Daniel,
- Jørgen Jensen

Abstract
Intake of protein immediately after exercise stimulates protein synthesis but improved recovery of performance is not consistently observed. The primary aim of the present study was to compare performance 18 h after exhaustive cycling in a randomized diet-controlled study (175 kJ·kg-1 during 18 h) when subjects were supplemented with protein plus carbohydrate or carbohydrate only in a 2-h window starting immediately after exhaustive cycling. The second aim was to investigate the effect of no nutrition during the first 2 h and low total energy intake (113 kJ·kg-1 during 18 h) on performance when protein intake was similar. Eight endurance-trained subjects cycled at 237±6 Watt (~72% VO2max) until exhaustion (TTE) on three occasions, and supplemented with 1.2 g carbohydrate·kg-1·h-1 (CHO), 0.8 g carbohydrate + 0.4 g protein·kg-1·h-1 (CHO+PRO) or placebo without energy (PLA). Intake of CHO+PROT increased plasma glucose, insulin, and branch chained amino acids, whereas CHO only increased glucose and insulin. Eighteen hours later, subjects performed another TTE at 237±6 Watt. TTE was increased after intake of CHO+PROT compared to CHO (63.5±4.4 vs 49.8±5.4 min; p<0.05). PLA reduced TTE to 42.8±5.1 min (p<0.05 vs CHO). Nitrogen balance was positive in CHO+PROT, and negative in CHO and PLA. In conclusion, performance was higher 18 h after exhaustive cycling with intake of CHO+PROT compared to an isocaloric amount of carbohydrate during the first 2 h post exercise. Intake of a similar amount of protein but less carbohydrate during the 18 h recovery period reduced performance.
Citation: Rustad PI, Sailer M, Cumming KT, Jeppesen PB, Kolnes KJ, Sollie O, et al. (2016) Intake of Protein Plus Carbohydrate during the First Two Hours after Exhaustive Cycling Improves Performance the following Day. PLoS ONE 11(4): e0153229. https://doi.org/10.1371/journal.pone.0153229
Editor: Nir Eynon, Victoria University, AUSTRALIA
Received: November 10, 2015; Accepted: March 27, 2016; Published: April 14, 2016
Copyright: © 2016 Rustad et al. This is an open access article distributed under the terms of the Creative Commons Attribution License, which permits unrestricted use, distribution, and reproduction in any medium, provided the original author and source are credited.
Data Availability: The project has no approval from Regional Committees for Medical and Health Research Ethic, Norway for public deposition of the original data, and participants give no approval in signed consent form for public deposition of data. All data are from the “Intake of protein plus carbohydrate during the first two hours after exhaustive cycling improves performance the following day” study are available on request. JJ has all data and may be contacted at jorgen.jensen@nih.no.
Funding: The study was funded by Norwegian School of Sport Sciences.
Competing interests: The authors have declared that no competing interests exist.
Introduction
Insufficient recovery of performance capacity after exhaustive endurance exercise may determine the final outcome in cycling competitions like the Tour de France with consecutive racing days. It is well-documented that muscle glycogen is the major energy substrate during high intensity endurance exercise and it is also known that fatigue develops when muscle glycogen is depleted [1–4]. A high glycogen content in muscles is thus associated with improved performance [5] and a repletion of glycogen stores is part of the recovery process after prolonged exhaustive exercise [6]. Optimal glycogen repletion occurs with an intake of 1.2 g carbohydrate · kg-1 · h-1 during the first hours and a total of 8–9 g carbohydrate · kg-1 during 24 h, which is now accordingly recommended [7,8].
Protein is normally not considered as an important energy substrate during exercise, although oxidation of leucine is known to increase during exercise [9,10]. Furthermore, protein degradation is increased in glycogen-depleted muscles during exercise [11–13], and protein breakdown continues in skeletal muscle after exercise if energy intake is not sufficient or if only carbohydrates are provided [14]. Recovery of performance includes the removal and degradation of proteins damaged during exercise along with the proper resynthesis of proteins.
Dietary intake of branched chain amino acids (BCAA) after exercise reduces loss of amino acids from muscles and therefore spares muscle protein from degradation [15]. Intake of protein immediately after exercise has also consistently shown to increase protein synthesis [16,17], including synthesis of mitochondrial proteins [18], while simultaneously protein degradation is decreased [17]. Importantly, it has been shown that protein intake in the early phase after intensive exercise stimulates protein synthesis more effectively than later [19]. When intake of proteins is combined with carbohydrate after exercise, activation of hypertrophic signalling molecules in skeletal muscles is achieved [20]. Protein intake is necessary for optimal recovery after exhaustive exercise [21,22] requiring both glycogen and protein synthesis [22]. Moreover, there is evidence that the combination of carbohydrates with protein also increases the rate of glycogen synthesis [21,23–25].
Despite the proven effects of protein intake on protein synthesis rate, supplementation of proteins in addition to carbohydrates is not consistently associated with improved exercise performance. Several studies have reported that intake of protein after exercise improves subsequent performance [20,21,26–29] whereas other studies failed to observe such effects [30–33].
These discrepancies may result from differences in the amount of protein supplied, its composition or that the exercise models used may not be adequate to detect the effects of protein intake on recovery of performance. Quite different protocols regarding intensity and duration prior to the diet intervention, and to assess performance after the intervention period have been used. An experimental design with similar exercise intensity prior to and after the diet intervention would represent a more appropriate approach for getting more coherent outcomes assuming that the physiological mechanisms causing fatigue during the initial bout of exhaustive exercise would limit performance capacity during the post exercise test.
In the present study, subjects were exercised to exhaustion at a load corresponding to ~72% VO2max (Watt72%), which depletes glycogen stores and such exercise has been used to demonstrate a positive effect of protein intake on recovery of performance [21,26]. The primary aim of the present study was to test the hypothesis that protein/carbohydrate feeding during the first 2 hours after exhaustive exercise is superior to intake of an isocaloric amount of carbohydrate alone on recovery of performance 18 h later. Therefore, we performed a randomized, double-blinded, diet-controlled study in which subjects were given carbohydrate (1.2 g · kg-1 · h-1) or protein/carbohydrate (0.4 g/0.8 · kg-1 · h-1) during the first 2 h after exhaustive exercise with diet during the remainder of the recovery period standardized. Nitrogen balance, plasma glucose, amino acids and hormones were measured during recovery and an exercise performance test conducted the following day to determine whether changes in performance after protein intake immediately after exhaustive exercise are associated with distinct changes in energy and amino acid homeostasis.
To test the sensitivity of our protocol selected for investigating exercise performance recovery, we also investigated the effect of insufficient energy intake during recovery, which incompletely restores glycogen content [34]. Therefore, the second aim was to investigate if lack of energy intake during the first 2 h after exhaustive endurance exercise, and a ~50% reduction of total carbohydrate intake, reduces exercise performance after the 18 h recovery period.
Materials and Methods
Subjects
Eight male endurance-trained subjects using cycling as part of their weekly training participated in the study (age: 24±0.4 years; height: 182±3 cm; weight 75±3 kg; cycle training 2.9 ±0.6 h weekly). Maximal oxygen uptake was 5.2 ± 0.1 L · min-1 (69.6 ±1.3 ml · kg-1 · min-1). The study was approved by the Regional Committees for Medical and Health Research Ethics (2010/1537/REK sør-øst D), Norway. Participants obtained verbal and written information about the study and signed informed consent to participate. Informed consent was approved by ethics committee and signed forms are stored.
Experimental design
The subjects completed three performance tests after three different diet interventions supplied after bouts of exhaustive exercise. The study was double-blinded and randomized with a balanced crossover design. The interventions were separated by a minimum of 6 days. The protocol is described in Fig 1.
Initial tests.
During the first day of the study, subjects performed an incremental test on a cycle ergometer (Lode Excalibur, Lode, Groningen; The Netherlands) at which time the relationship between work (Watt) and oxygen uptake (VO2) was established. Subjects started at 100 or 125 W (Mean 109.4 ± 6.6 W) and the load was increased by 25 Watt every 5 min. The final intensity was 237.5 ± 9.4 W. VO2 was measured on-line (Oxycon Pro; Jager Instruments, Hoechberg, Germany) over 1 min (from ~2.5–4 min at each intensity level) and capillary blood samples were taken for lactate analysis (1500 Sport, YSI Inc., Yellow Spring Instruments Ohio, USA). The incremental test was terminated when the lactate concentration had increased > 2 mM. The mean lactate concentration at the final intensity level was 2.4 ± 0.1 mM. Subjects selected a pedaling frequency between 85 and 95 rpm, and the self-selected pedaling frequency was used throughout the study. After 5 min of rest, maximal oxygen uptake (VO2max) was measured. Subjects started the VO2max test at the highest (last) load of the incremental test and intensity was increased by 25 W every 30 s until voluntary exhaustion. Maximal lactate concentration averaged 8.2 ± 0.3 mM and RER was 1.11 ± 0.02 at the end of the VO2max test. VO2 was measured over 30 s periods, and the mean of the two highest measurements was defined as maximal oxygen uptake (VO2max). The subjects returned to the laboratory 2 to 4 days after the test of VO2max to complete a pretest in which subjects cycled 45 min at a load corresponding to ~72% of VO2max. This workload was calculated from the first incremental test (linear regression was used to establish the relationship between work and VO2). VO2 was measured between 3–5 min and the workload was adjusted to achieve a VO2 corresponding to ~72% of VO2max (Watt72%). The defined load (Watt72%) was used in all tests at both day1 and day2.
Diet interventions
Subjects recorded their dietary intake over the last 24 h prior to the first intervention period and were asked to follow the same diet prior to the two other interventions. No exercise was allowed for a minimum of 24 h before the exhaustive bout of exercise before the dietary interventions. Before the three diet intervention phases (Day1), subjects completed an exercise block with cycling until exhaustion. Subjects reported to the laboratory at 13.30 h and prepared for exhaustive bouts of exercise starting at 14.00 h (Fig 1). The training session was initiated with a 3 x 4 min warm up at 50, 55 and 60% of VO2max. Subjects then cycled at Watt72% until exhaustion in 20 min intervals with 5 min rest between intervals. Exhaustion was defined as when subjects were unable to maintain the desired pedal frequency (self-selected pedal frequency ± 5 rpm) at the desired workload despite the third verbal encouragement to maintain the pedal frequency. After voluntary exhaustion, subjects were given 5 min of rest before completing a maximal number of 1 min intervals at 90% of VO2max with 1 min rest between intervals. This protocol has been reported to reduce glycogen level in m vastus lateralis to a very low level [1]. VO2 and RER were measured after 4 min of the first interval and during the last 2 min of all intervals for calculation of carbohydrate oxidation. Capillary blood samples were taken before the first 20 min interval, immediately after each interval, at exhaustion and immediately after the last 1 min interval for measurements of lactate (described above) and glucose (HemoCue Glucose 201+, Ängholm, Sweden). Heart rate was measured continuously (Polar RS800CX, Kempele, Finland).
After the exhaustive exercise session, a catheter venflon was inserted into antecubital vein and a blood sample (7 ml) was taken. Subjects then received the first drink. For the first two hour of the diet interventions the subjects received a drink containing either: 1) 1.2 g carbohydrate (CHO) · kg-1 · h-1, 2) 0.8 g CHO · kg-1 · h-1 and 0.4 g whey · kg-1 · h-1 (CHO+PROT) or 3) similar flavored placebo without energy (PLA) in random order. The drink was supplied in equal portions every 30 min to obtain the energy level described. The CHO drink contained 85 g/L of glucose (Merck KGaA (Darmstadt, Germany) and 85 g/L maltodextrin (Carbo Flex Pure, Star Nutrition, Gymgrossisten Trollhättan, Sweden). CHO+PROT drink contained 56.5 g/L glucose and 56.5 g/L maltodextrin and 57 g/L whey isolate protein (Lacprodan, SP-9225 Instant, Arla, Aarhus, Danmark). PLA was water. All drinks contained 0.7 g/L NaCl and were flavored by 100 g/L Fun light (Stabburet, Norway). Drinks were served in opaque flasks and were indistinguishable by taste.
The first blood sample was taken before drink consumption, and capillary and venous blood samples were taken after15, 30, 60, 90 and 120 min of the recovery period as described above. Resting metabolic rate was measured after the blood samples were taken at 30, 60, 90 and 120 min of recovery according to the following protocol: subjects rested on a bed for 10 min before a V2 mask was used to collect air for 10 min.
After the first 2 h of the recovery period (and RER measurements) subjects had a standardized meal (composed of pasta, grained meat and tomato sauce). Energy content of the meal (and all other meals) was adjusted to body weight with macro nutrient content of: carbohydrate, 2.08 g · kg-1; protein, 0.59 g · kg-1; fat, 0.27 g· kg-1 (55.4 kJ ·kg-1). The subjects returned to their homes with additional drink and evening food. During the CHO and CHO+PROT trials, subjects received a drink containing 1.2 g CHO · kg-1 at 19.30 h, whereas PLA received flavored drink without energy. At 21.30 h, subjects had sandwiches with the following energy content: carbohydrate, 0.82 g · kg-1; protein, 0.27 g · kg-1; fat, 0.27 g· kg-1 (28.5 kJ ·kg-1). Water was allowed ad libitum. Subjects were not allowed to eat food other than that provided.
The following morning, subjects reported to the laboratory at 7.30 h and resting metabolic rate was measured. Subjects were not allowed to do any exercise for transportation to the laboratory, and rested 10 min in the bed before resting metabolic rate was measured as described. Fasted venous and capillary blood samples were taken and subjects received a light breakfast at 8 h. The breakfast had the following energy content: carbohydrate, 1.05 g · kg-1; protein, 0.17 g · kg-1; fat 0.18 g· kg-1 (27.4 Total kJ ·kg-1). During the diet intervention CHO and CHO+PROT were isocaloric (175 kJ · kg-1), but protein intake during the 18 h of recovery was 1.03 g · kg-1 and 1.83 g · kg-1 in CHO and CHO+PROT, respectively. The CHO and PLA interventions had the same intake of protein (1.03 g · kg-1) during the 18 h intervention, but carbohydrate intake was 7.55 g · kg-1 and 3.95 g · kg-1 in CHO and PLA, respectively. The study was designed with similar total energy intake in CHO and CHO+PRO during the 18 h recovery. Protein intake was higher in CHO+PROT (1.83 g · kg-1) than in CHO (1.03 g · kg-1) with carbohydrates substituted for protein in CHO (S1 Table). Protein intake was the same in CHO and PLA, but carbohydrate intake was reduced by 48% in PLA compared to CHO. Total energy intake during the 18 hour long recovery period was 13073 ± 483, 13073 ± 483 and 8441 ± 312 kJ in CHO, CHO+PROT and PLA, respectively.
Performance test
The subjects reported to the laboratory at 7.30 h and resting metabolic rate was measured as described above. A fasting blood sample was taken before a standardized breakfast was served. A venflon was inserted into an antecubital vein at 9.30 h and subjects prepared for the performance test. The performance test was defined as cycling until exhaustion at Watt72%. The performance tests started approximately after 18 h of recovery and dietary intervention (Day2). Warm up started at 9.45 h and consisted of 3 x 4 min at 50, 55 and 60% of VO2max. The performance test started at 10.00 h and subjects cycled at Watt72% (the same load as the day before corresponding to ~72% of VO2max) until exhaustion. Capillary and venous blood samples were taken before the performance test, after 15, 30 min, and every 30 min during the performance test, at exhaustion, and 15 and 30 min after exhaustion. VO2 and RER were measured after 4, 15, 30 min and thereafter every 30 min. VO2 and RER were also measured during the last min of exercise. Heart rate was recorded continuously during the performance test. Subjects reported rate of perceived exertion according to the Borg scale after 2, 15, 30 min and thereafter every 30 min. Subjects also reported rate of perceived exertion at exhaustion.
Blood analyses
Venous blood samples (7 ml) were taken in tubes containing 140 μl 3 mM EGTA/2.5 mM glutathione in reduced form. Immediately thereafter, 2 ml of blood was transferred to another tube, which contained Aprotinin (500 KIE/ml) for measurement of glucagon and insulin. Blood samples were kept on ice until centrifugation (2500 g; 10 min; 4°C), and plasma was stored at -70°C until analysis. All analytic processes were conducted according to the manufacturer’s instructions.
Plasma glucose.
Plasma glucose content was determined applying an enzymatic reference method GLUC assay (Roche Diagnostics GmbH, Mannheim, Germany) on a Cobas c111 system (Roche, Germany).
Insulin.
Plasma insulin concentrations were measured with an enzyme-linked immunosorbent assay human insulin kit, K6219 (Dako, Glostrup, Denmark).
Glucagon.
Plasma glucagon concentrations were determined by a radioimmunoassay (RIA) kit (EMD Millipore Corporation, Billerica, MA, USA).
Free Fatty Acids (FFA).
The FFA content was measured by an in vitro enzymatic colorimetric assay for the quantitative determination of non-esterified fatty acids (NEFA-HR) (Wako Chemicals GmbH, Neuss, Germany) using the autoanalyzer Cobas C-111 (Roche, Germany).
Glycerol.
The glycerol content was measured by a direct colorimetric procedure (glycerol Randox, Crumlin, UK) using the autoanalyzer Cobas C-111 (Roche, Germany).
Creatine kinase.
The Creatine Kinase (CK) content was measured by Siemens Dimension® CKMB Creatine Kinase Isoenzyme MB assay, Creatine Kinase assay (Siemens, Newark, USA) and analyzed on a Dimension Vista 1500 (Siemens Healthcare GmbH, Erlangen, Germany).
Lactate Dehydrogenase.
The content Lactate Dehydrogenase (LDH) was measured by a Lactate Dehydrogenase assay kit (Siemens, Newark, USA) and analyzed on a Dimension Vista 1500 (Siemens Healthcare GmbH, Erlangen, Germany).
Myoglobin.
Myoglobin content was determined by immunoassay for the in vitro quantitative determination in human plasma, Myoglobin Assay (Roche Diagnostics GmbH, Mannheim, Germany), and analyzed on a Cobas 6000 E-modul (Roche Diagnostics GmbH, Germany).
Amino acids.
For quantification of amino acids a targeted LC-MS/MS approach with iTRAQ® (AA45/32™ Phys REAG Kit, Applied Biosystems, USA) labeling was used as described previously [35]. Plasma sample preparation was performed according to the manufacturer's instructions with 40 μl of sample volume.
Calculation of carbohydrate and fat oxidation.
Carbohydrate (and fat) oxidation was calculated from RER and oxygen uptake according to Peronnet and Massicotte [36] as non-protein metabolism.
Urine urea and creatinine.
Urine was collected during the recovery in three time periods (0–2 h; 2–8 h and 8–16 h) as shown in Fig 1. From the three periods, total volume was estimated and a 20 ml sample frozen at– 20°C for analysis of urea and creatinine. Urea and creatinine were determined on a MaxMat PL auto analyser (MaxMat PL, Montpellier, France) with a MaxMat urea kit (RM UREE0252) and a MaxMat creatinine PAP kit (RM CREP0270) according to instructions.
Calculation of nitrogen balance.
Nitrogen balance was calculated from protein and nitrogen excretion over the 18 h recovery period. Nitrogen intake was calculated assuming the nitrogen to amino acid constant of 6.5 [37]. Nitrogen excretion was calculated from urea excretion as described by Rowlands et al. [29].
Motivation and rate of perceived exertion (RPE).
Motivation was rated on the 0–100 scale (from “no focus” to “perfect focus”) described previously [38]. RPE was rated on the 6–20 Borg scale [39].
Statistics.
Data are presented as the mean±SEM. Comparisons of performance and nitrogen balance among treatments were performed with ANOVA and Fishers LSD was used as a post hoc test. Repeated measurements MANOVA were used to test responses in hormones, metabolites, and amino acids during the recovery period. Because time to exhaustion differed between subjects as well as diet, hormone, metabolites and amino acid responses at the 6 data points, blood samples (morning, before exercise, 15 min of exercise, exhaustion, and 15 min and 30 min after exercise) were evaluated by repeated measurements MANOVA. Variables are considered significant when p<0.05.
Results
Exhaustive exercise before the diet interventions (Day 1)
Subjects standardized their diet and exercise the last 24 hours before the bouts of exhaustive exercise prior to the dietary interventions (Day1); questionnaires confirmed adherence to instructions. Subjects cycled at 237 ± 6 Watt (Watt72%) and exhaustion occurred after 89.5 ± 10.1, 82.4 ± 7.8 and 87.3 ± 8.0 min prior to CHO, CHO+PROT and PLA, respectively. There was no difference in total cycling time before the three diet interventions. Mean oxygen uptake during the exhaustive exercise was 3.8 ± 0.1 L∙ min-1, which corresponded to 72.2 ± 1.1% of VO2max, during the exhaustive cycling at Watt72%. The participants completed 5 ± 1 1-min sprints at 90% of VO2max after 5 min rest (after exhausting cycling); the number of sprints did not differ before the three interventions. Oxygen consumption increased slightly whereas RER decreased slightly during the exhaustive exercise at Watt72% (Table 1). Total carbohydrate oxidation during the exhaustive cycling was 261 ± 28, 233 ± 22 and 226 ± 21 g before the CHO, CHO+PROT and PLA, respectively, and did not differ. Blood glucose decreased gradually during exercise and was 3.0 ± 0.1 mM at exhaustion (Table 1). Blood lactate rose from 0.73 ± 0.05 mM to about ~2.0 mM during the first 20 min interval and remained stable thereafter (Table 1). There were no differences in lactate responses during the exhaustive exercise prior to the three dietary interventions.
Recovery period
Blood lactate concentrations decreased rapidly after exhaustive exercise independent of dietary intervention. Lactate levels declined rapidly and were back at baseline after 30 minutes in all three dietary interventions (Fig 2A). Blood glucose concentration increased rapidly after intake of CHO and CHO+PROT (Fig 2B). At 60 and 90 min the blood glucose concentration was significantly higher after intake of CHO compared to CHO+PROT (Fig 2A). In PLA blood glucose remained low (~3.5 mM) during the 2 h of recovery after the exhaustive bout of exercise and was significantly lower than CHO and CHO+PROT. Insulin concentration rose after ingestion of CHO and CHO+PROT, but not in PLA (Fig 2C). There was a tendency for a higher insulin concentration at 60 minutes (p = 0.053) after ingestion of CHO+PROT as compared to CHO alone. Area under curve also tended to be higher for insulin after CHO+PROT compared to CHO (p<0.06). FFA concentration decreased rapidly after intake of CHO and CHO+PROT, but remained elevated after PLA (Fig 2D). Plasma glycerol decreased rapidly after exhaustive exercise but was higher in PLA than CHO and CHO+PROT in the 60–120 min period (Fig 2E). Glucagon concentration decreased after exhaustive exercise, but more so in CHO and CHO+PROT than in PLA (Fig 2F).
Data are mean and error bars represents SEM. C: p<0.05 compared to CHO; W: p<0.05 compared to CHO+PROT (whey); P: p<0.05 compared to placebo. N = 8 for all data points.
https://doi.org/10.1371/journal.pone.0153229.g002
VO2 and RER where determined every 30 min during the first 2 h of recovery and used to calculate carbohydrate oxidation rate (S2 Table). RER values were suppressed below 0.70 during the first 30 min of recovery in each of the treatments. RER increased during intake of CHO and CHO+PROT, but RER remained below 0.70 in PLA during the first 2 h of recovery. VO2 was higher after intake of CHO+PROT as compared to CHO and PLA.
Concentrations of amino acids during the three interventions are shown in Fig 3. Plasma concentrations of many amino acids including BCAA increased after intake of CHO+PROT whereas their concentrations remained unchanged in PLA (Fig 3A–3C). In contrast, the concentrations of BCAA decreased during the 2 h CHO supplementation, most likely due to increased BCAA clearance resulting from an increase in plasma insulin. The concentration of the aromatic amino acids (phenylalanine and tyrosine), which are essentially not degraded in skeletal muscle, remained stable in PLA. In CHO+PROT, concentrations of several amino acids increased (arginine, proline, asparagine, serine, threonine, lysine and tryptophan) while remaining stable during PLA (Fig 3). Amino acid concentrations at exhaustion did not differ among exhaustive exercises prior to the three dietary interventions. Mean concentrations of plasma amino acids at exhaustion are shown in S3 Table.
Data for 18 amino acids are presented; no data are presented on cysteine and aspartate. Data are mean and error bars represents SEM. C: p<0.05 compared to CHO; W: p<0.05 compared to CHO+PROT (whey); P: p<0.05 compared to placebo. N = 8 for all data points.
https://doi.org/10.1371/journal.pone.0153229.g003
Uric acid increased gradually during the recovery period and was higher in PLA compared to CHO and CHO+PROT after 60 min (Fig 4A). Concentrations of ornithine increased in CHO+PROT, but remained stable in CHO and PLA (Fig 4B). Data on other amino and organic acids are shown in Fig 4. Citrulline remained stable during intake of CHO+PROT, but decreased in CHO and PLA, and more in CHO than in PLA. Dietary intervention had no effect of plasma 1-methyl-histidine during the first 2 h of recovery.
Data are mean and error bars represents SEM. C: p<0.05 compared to CHO; W: p<0.05 compared to CHO+PROT (whey); P: p<0.05 compared to placebo. N = 8 for all data points.
https://doi.org/10.1371/journal.pone.0153229.g004
Fasted values the following morning (Day 2)
Fasting blood glucose levels were significantly higher after ingestion of CHO and CHO+PROT compared to PLA (p<0.05; Table 2) the morning after the initial exhaustive exercise. RER and CHO oxidation was significantly higher in CHO compared to PLA (p<0.05), but there was no difference between CHO and CHO+PROT. Fasting insulin, FFA, glycerol and glucagon levels did not differ between diet interventions.
Fasting concentrations of most amino acids (asparagine, glutamine, glycine, histidine, isoleucine, leucine, lysine, phenylalanine, proline, serine, threonine, tryptophan) were increased similarly in CHO+PROT, CHO and PLA compared to values immediately after exhaustive exercise the day before (S3 Table). Fasting morning concentrations of lysine, proline and threonine were higher after CHO+PROT compared to both CHO and PLA. Concentration of valine increased only in CHO+PROT and PLA, and was lower in CHO compared to CHO+PROT and PLA. Concentration of alanine decreased significantly compared to immediately after exhaustion Day 1, with no difference among dietary interventions. Plasma organic acids are shown in S4 Table.
Time to exhaustion (TTE) at the performance tests the day after the dietary interventions
For the performance test after the interventions (Day2) time to exhaustion cycling at Watt72% (237 ± 6 W) was longer after CHO+PROT compared to CHO (63.5 ± 4.4 versus 49.8 ± 5.4 min; p<0.05) (Fig 5). For PLA, time to exhaustion was 42.8 ± 5.1 min, which was lower than CHO (p<0.05). Noteworthy is our observation that cycle time was longer in all test subjects after ingestion of CHO+PROT compared to CHO and PLA. However, prior to the dietary interventions (Day1) participants also cycled to exhaustion at Watt72% (237 ± 6 W), but in intervals of 20 min with 5 min rest between intervals.
The performance test was time to exhaustion cycling at W72% (237±6 W). Data are mean and error bars represents SEM. N = 8 at all tests. a: p<0.05 compared to CHO+PROT; b: p<0.05 compared to CHO.
https://doi.org/10.1371/journal.pone.0153229.g005
Metabolic data during performance tests (TTE) the day after the dietary interventions (Day2)
Oxygen uptake during the TTE (237 ± 6 W) was similar after all diet interventions and the oxygen uptake during Day2 corresponded to 73.2 ± 0.9% of VO2max. RER and carbohydrate oxidation during the TTE were significantly lower in PLA after 4 and 15 min compared to CHO (p<0.01; Table 3). There was no difference between CHO and CHO+PROT at the start of TTE. After 15 min, RER and carbohydrate oxidation were significantly lower after ingestion of PLA compared with CHO and CHO+PROT (p<0.01). Total carbohydrate oxidation during TTE was 136 ± 14, 157 ± 13 and 96 ± 11 g after ingestion of CHO, CHO+PROT and PLA, respectively (Table 3). Total carbohydrate oxidation was significantly higher after ingestion of CHO+PROT compared to CHO (p<0.05) and PLA (p<0.01) and CHO compared to PLA (p<0.01).
Lactate increased during the TTE and there were no differences at any time point after the three dietary interventions (Fig 6A). Fasting glucose was lower in PLA compared to CHO (Fig 6B). During TTE the blood glucose concentration declined significantly in each treatment. The blood glucose concentration was significantly lower after 15 min of cycling after ingestion of PLA compared to CHO and CHO+PROT (p<0.05; ANOVA comparison at time point). There were no differences in glucose concentration at exhaustion among treatments. Fasted plasma insulin was low and similar between diet interventions, and increased similarly after breakfast on Day2 (Fig 6C). During the TTE, plasma insulin dropped to low levels in all treatments.
Data are mean and error bars represents SEM. C: p<0.05 compared to CHO; W: p<0.05 compared to CHO+PROT (whey); P: p<0.05 compared to placebo. N = 8 for all data points.
https://doi.org/10.1371/journal.pone.0153229.g006
The plasma FFA responses during the TTE were different among the diet interventions. Interestingly, plasma FFA was lower in CHO compared to PLA after 15 min of exercise (Fig 6D). Furthermore, after the TTE, plasma FFA increased to higher levels in CHO+PROT and PLA compared to CHO. Plasma glycerol also responded differently during TTE after the dietary interventions (Fig 6E), and suggested that lipolysis was reduced in CHO compared with CHO+PROT. Plasma glucagon responses during the TTE differed after the dietary interventions, and the results may be of importance for understanding the lower time to exhaustion in CHO. Interestingly, glucagon did not increase significantly during the TTE in CHO (Fig 6F) and was higher in PLA and CHO+PROT at exhaustion.
During TTE oxygen uptake increased with all dietary interventions, but the increase was faster in PLA compared to CHO (Table 3). RER (and carbohydrate oxidation) decreased gradually during the TTE with RER being lower for PLA compared to CHO and CHO+PROT after 15 min of exercise (Table 3). Heart rate increased gradually after all interventions, but was higher at exhaustion in PLA compared to CHO and CHO+PROT (Table 3).
Amino acids
The concentrations on most amino acids were similar before the TTE among treatments (Fig 7). However, the concentration of valine was lower in CHO compared to CHO+PROT and PLA (Fig 7C), whereas the concentrations of threonine and lysine were higher after CHO+PROT compared to CHO and PLA (Fig 7P and 7Q). Concentration of tryptophan was lower in PLA compared to CHO after exercise. During the TTE all amino acids responded similarly among treatments except valine, which remained lower in CHO compared to PLA (Fig 7C). Interestingly, glutamate decreased significantly during TTE under all dietary interventions (Fig 7F). There were no differences in responses in ornithine and citrulline during the TTE after the three dietary interventions (S1 Fig). Taurine increased during the TTE after all dietary interventions (S1 Fig).
Data are mean and error bars represents SEM. C: p<0.05 compared to CHO; W: p<0.05 compared to CHO+PROT (whey); P: p<0.05 compared to placebo. N = 8 for all data points.
https://doi.org/10.1371/journal.pone.0153229.g007
Nitrogen balance
Urine sampling was initiated at the end of the exhaustive exercise prior to the dietary intervention (Day 1) until the following morning; the day of the performance test. Total nitrogen excretion was higher in CHO+PROT compared to CHO and PLA (Fig 8A). Nitrogen balance was positive in CHO+PROT and negative in CHO and PLA (Fig 8B) with nitrogen balance more negative in PLA compared to CHO (Fig 8B). Creatinine excretion was similar during the three diet interventions (Fig 8C). Plasma uric acid remained higher after PLA the following morning and remained higher than CHO and CHO-PROT during the TTE (S1 Fig).
Data are mean and error bars represents SEM. C: p<0.05 compared to CHO; W: p<0.05 compared to CHO+PROT (whey); P: p<0.05 compared to placebo. N = 8 for all data points.
https://doi.org/10.1371/journal.pone.0153229.g008
Muscle damage
Plasma CK, LDH and myoglobin were measured as markers of muscle damage. The dietary interventions did not influence the markers for muscle damage after exhaustive cycling (S5 Table).
Motivation and rate of perceived exertion
On a scale from 0–100 the motivation to perform during TTE was 76 ± 4, 80 ± 3 and 84 ± 3 after ingestion of CHO, CHO+PROT and PLA. These numbers correspond to high motivation to perform maximally, and there were no differences in motivation among the three dietary interventions. There also were no differences in rating of perceived exertion among the three dietary interventions at the beginning of the TTE (Fig 9). However, at 15 min of cycling rating of perceived exertion was significantly higher after ingestion of PLA compared to CHO+PROT and CHO (p<0.05). RPE at exhaustion was similar during the three performance tests (TTE).
Data are mean and error bars represents SEM. C: p<0.05 compared to CHO; W: p<0.05 compared to CHO+PROT (whey); P: p<0.05 compared to placebo. N = 8 for all data points.
https://doi.org/10.1371/journal.pone.0153229.g009
Discussion
The main finding in the present study is that a CHO+PROT supplementation during the first 2 h after exhaustive cycling was superior to an isocaloric carbohydrate supplement for cycling performance recover when assessed 18 h later. CHO+PROT supplementation immediately after exercise increased plasma concentrations of glucose, insulin, BCAA and a number of other amino acids. CHO supplementation also caused increases in plasma glucose and insulin, but the concentration of many amino acids decreased, probably caused by insulin effects on amino acid removal from plasma. Nitrogen balance during the recovery period was positive after intake of protein immediately after exhaustion (CHO+PROT), but was negative in CHO despite that 1.03 g protein ∙ kg body weight-1 was provided during the 18 h recovery. Reducing the intake of carbohydrates (PLA) during the recovery period resulted in a more negative protein balance and reduced recovery of performance compared to CHO. Our results suggest that CHO+PROT during the first 2 h of an 18 h recovery period accelerates repair of protein function and possibly resynthesis of muscle glycogen stores [6,23–25] resulting in improved exercise performance at the end of recovery. Limiting carbohydrate intake during recovery also adversely affected recovery, and this may be related to the inability to recover sufficient muscle glycogen stores [34].
In the present study cycling time to exhaustion after 18 h of recovery was longer after CHO+PROT supplementation compared to CHO, when the same workload (Watt72%) was used to induce exhaustion prior to dietary intervention and during the performance test the following day. The effect of CHO+PROT intake during the first 2 h after exhaustive exercise was convincing as all 8 subjects performed best after intake of CHO+PROT compared to both CHO and PLA. The findings of improved performance after ingestion of protein combined with carbohydrates immediately after exhaustion as compared to carbohydrates only are in line with those of several other studies using time to exhaustion as the performance test [21,26]. Saunders et al. also showed a dramatic effect of protein intake when subjects cycled to exhaustion at 75% VO2max and 16 h later used cycling to exhaustion at 85% VO2max to test recovery of performance [26]. Moreover, Ferguson-Stegall et al. found a significant increase in cycling time trial performance after 4 h of exercise recovery when a CHO+PRO supplement was provided as compared with an isocaloric CHO supplement or placebo [20]. However, co-ingestion of protein with carbohydrate after exercise has not always been found to have a positive effect on recovery of performance [31,40]. Furthermore, Rowlands et al. found a positive effect of protein supplementation post exercise on recovery of performance in some [28
Feed intake of the sow and playful creep feeding of piglets influence piglet behaviour and performance before and after weaning
Abstract
Creep feed intake is variable and may be partly homeostatically and exploratory driven. We studied effects of maternal feed restriction and a ‘play-feeder’ on piglet behaviour and performance. 37 Litters received creep feed in a conventional (CON) or play-feeder (PL) and their sows were full-fed (FF) or restrictedly-fed (RES). Eaters were determined via rectal swabs. At weaning (d24) four piglets from the same treatment were grouped (n = 36 pens). RES hindered piglet growth by 41 g/d and enhanced time eating, creep feed intake and percentage of eaters at weaning versus FF. RES-PL had the largest proportion of moderate and good eaters. PL stimulated feeder exploration and attracted more piglets to the feeder than CON. Post-weaning, RES increased exploratory behaviours, feed intake between d0–5, and growth between d0–2, and reduced body lesions between d0–2 (within CON), drinking and ear biting. PL increased ingestive behaviours, feed intake and growth between d0–15, and BW at d15 post-weaning by 5%. PL also lowered the prevalence of watery diarrhoea, number of body lesions and piglets with ear (within FF) and tail (within RES) damage at d15 post-weaning. Treatments did not affect FCR. To conclude, RES and particularly PL (broader and for longer) result in less weaning-associated-problems.
Introduction
The transition from feeding on sow’s milk to foraging and feeding exclusively on solid feed, i.e. weaning, is a gradual process occurring between 8.5 and 22 weeks of age in free range pigs1,2. In commercial farms, weaning occurs earlier and sudden by separation of the sows and their piglets, generally at 3 to 4 weeks of age in Europe. Apart from this nutritional challenge, as piglets are abruptly not able to suckle anymore, weaning also simultaneously involves social (maternal separation and mixing with unfamiliar peers) and environmental stressors (handling, transport and housing in a novel pen). These stressors together often lead to a low feed (energy) intake (reviewed by3), gastro-intestinal problems (reviewed by4), gut microbiota dysbiosis (reviewed by5), reduced growth and behavioural disturbances6,7 after weaning, thereby reducing health and welfare. Stimulating the consumption of solid feed prior to weaning, i.e. creep feed, may improve piglet adaptation after weaning8,9,10,11, but factors influencing creep feed intake in piglets are unclear up to now.
Based on the findings by Algers et al.12, it was suggested that energy availability from milk affects individual creep feed intake. The proposed ‘compensatory feeding hypothesis’ suggests that slower-growing piglets in the litter will compensate for a lower energy intake from milk by consuming more creep feed and therefore undergo a less severe nutritional challenge at weaning and grow faster after weaning. Piglets classified as ‘good eaters’ of creep feed or with a long time (eating) at the feeder before and after weaning were, indeed, slower-growing piglets before weaning12,13,14,15. Complementary, other data suggest that the compensatory feeding hypothesis also holds on litter level, which may partly explain the large between-litter variation in creep feed intake8,9. Firstly, litters from intermittent suckling regimes that experienced reduced growth, due to separation from the sow for multiple hours per day, had a higher creep feed intake16,17, post-weaning feed intake10,18 and post-weaning weight gain19 than faster-growing conventional litters. However, effects of a low energy intake from milk are confounded in intermittent suckling regimes by the temporal maternal separation before weaning, which may increase separation stress before weaning, but reduce separation stress after weaning20. Secondly, primiparous sows, that produce less milk21,22,23 and consequently wean lighter piglets14,24, have been found to rear more eaters of creep feed than second parity sows, and particularly more moderate eaters of creep feed compared to multiparous sows14. Results of other studies, however, do not support or are even contradictory to the compensatory feeding hypothesis25,26,27.
It is likely that the consumption of creep feed is not solely driven by milk availability, as the milk production by the sow already becomes limiting for piglet growth from one week of lactation28 and a substantial proportion of piglets do not consume creep feed before they are weaned at 3 to 4 weeks of lactation10,11,27. Several studies suggested that creep feed intake may also be driven by intrinsic exploration in piglets towards the feed(er)29,30,31,32. Exploratory behaviour is closely related to play behaviour as both are likely involved in ‘object play’ in piglets, which includes holding or carrying an object or material in the mouth, manipulating, shaking and tugging it33. Object exploration is often hard to distinguish from and precedes object play (reviewed by34). In addition, exploratory behaviour, e.g. nosing, rooting, chewing, and object play seem to follow a similar developmental pattern in piglets. Both initiate in the first week of life and peak around four weeks of age, during which ingestion of solid feed items starts to increase35,36. It is therefore possible that exploratory behaviour and object play are involved in the development of feed intake behaviour in suckling piglets.
Our aim was to establish whether a low energy intake (homeostatic drive) from milk, by means of maternal feed restriction, and presenting creep feed in an exploratory and playful context (exploratory drive), by means of a foraging-stimulating play-feeder, are important and potentially interacting factors in getting piglets to eat creep feed. Subsequently, we studied the effects of the two factors on post-weaning adaptation in terms of behaviour and performance. We hypothesized that both factors would encourage piglets to familiarize themselves with creep feed and thereby reduce the stress from dietary changes at weaning and improve their adaptation after weaning.
Methods
The protocol of the experiment (sows: AVD104002015325, piglets: AVD104002016515) was approved by the Animal Care and Use committee of Wageningen University & Research (Wageningen, The Netherlands) and in accordance with the Dutch law on animal experimentation, which complies with the European Directive 2010/63/EU on the protection of animals used for scientific purposes. The use of Indigo carmine as feed colorant was approved by the Medicines Evaluation Board (Utrecht, The Netherlands).
Feeding strategies of sows and piglets during lactation
The study was set up as a 2 × 2 factorial arrangement with sow feeding (SF, lactational feed intake level) and piglet feeding (PF, creep feed presentation strategy) as experimental factors. All sows were fed the same level of commercially available sow diet twice a day (7:30 and 16:00 h) until d9 post-partum (8.9 MJ/kg as-fed net energy, 133 g crude protein, 5.6 standardized ileal digestible lysine/kg dry matter, ‘Standaard zeugenbrok’ during gestation and 9.3 MJ/kg as-fed net energy, 156 g crude protein, 7.7 standardized ileal digestible lysine/kg dry matter, ‘Zeugen Maxima Lacto’ during lactation, AgruniekRijnvallei, Wageningen, The Netherlands). The sows were provided 2.9 kg/d between d102–109 of gestation and the amount was gradually reduced to 2 kg/d at parturition, and increased thereafter with 0.5 kg/d. From d10 post-partum onwards, sows were fed in three feedings (7:00, 13:00 and 19:00 h) and received either 6.5 kg feed per day (full-fed, FF, n = 19) or 3.25 kg feed per day (restrictedly-fed, RES, n = 18) to create a contrast in energy supply from milk. The milk production of RES-sows was indeed lower compared to FF-sows, at least between d17–24 of lactation, and the milk of RES-sows contained a lower percentage of fat (Costermans et al., submitted). Moreover, RES-sows lost more body weight (19.2 ± 2.0 vs. 14.2 ± 2.0 kg) and muscle depth of the longissimus dorsi (1.3 ± 0.1 vs. 0.6 ± 0.1 mm) between d10–24 than FF-sows, but feed restriction of the sow did not affect back fat loss (3.4 ± 0.6 vs. 2.5 ± 0.6 mm)37. These changes were accompanied by lower plasma insulin-like growth factor 1 levels and higher plasma creatinine levels of RES-sows from d17 onwards, indicating a more severe negative energy balance of RES-sows37. A more detailed description of the effects of feed restriction during lactation on sow (reproductive) performance, energy mobilisation and milk quality are reported in Costermans et al.37 and Costermans et al. (submitted). Piglets reared by RES-sows will be named ‘RES-piglets’ and piglets reared by FF-sows will be named ‘FF-piglets’.
The litters were either creep-fed in a round conventional feeder (CON, n = 18 of which 9 FF and 9 RES; MS Clickfeeder Mini, MS Schippers, Bladel, The Netherlands) or in a foraging-stimulating ‘play-feeder’ (PL, n = 19 of which 10 FF and 9 RES) from d4 after birth. The piglet feeders had a diameter of 26 cm and five feeding places (Fig. 1a). The play-feeder was created by attaching canvas clothes (purchased at local market), braided natural cotton ropes (10 mm in diameter, MS Schippers, Bladel, The Netherlands) and PVC spiral tubes (13 mm in diameter, Ubbink, Alkmaar, The Netherlands) to the conventional feeder (Fig. 1b,c). These materials were attached on the inside at the bottom of the feeder (4 pieces of canvas clothes, 1 cotton rope, 1 PVC spiral tube). Two canvas clothes and one cotton rope were also tied to the feeder so they were hanging down and a PVC spiral ring was placed around the mounting system of the feeder so that it could be moved up and down. In this way the play-feeder allowed piglets to express object play as well as foraging behaviours, i.e. rooting, nosing, chewing, biting, pushing, pulling and lifting. A video showing interaction of piglets with the play-feeder can be found online as Supplementary Video S1. The canvas clothes and cotton ropes were replaced at d18. Feeders were located at the front end of the farrowing area near the feeding corridor.
Piglets were fed an experimental creep feed as crumble (Research Diet Services, Wijk bij Duurstede, The Netherlands). The creep feed (11.8 MJ/kg as-fed net energy, 195 g crude protein, 11.9 g standardized ileal digestible lysine/kg dry matter) was high in dietary non-starch polysaccharides (261 g/kg dry matter), originating from cereal grains, sugarbeet pulp, oat hulls, galacto-oligosaccharides, inulin and high-amylose starch (Supplementary Tables S2 and S3). Feed colorant Indigo carmine (E132 Eurocert 311811, Sensient Food Colors, Elburg, The Netherlands) was added manually to the pre-weaning piglet diet (5 g/kg of feed) to allow for identification of eaters (see below). Every morning a small scoop of fresh creep feed was given and feeders were checked at least twice daily and three times a day from d10 to provide the creep feed ad libitum. To maintain freshness of the creep feed, all feed was replaced and feed bowls were cleaned using paper towels and water at each feed weigh-back (see below). Two days prior to weaning, the creep feed was mixed with a commercially available crumbled nursery diet to familiarize piglets to the nursery diet before weaning (161 g crude protein, 48 g crude fibre and 11.8 g standardized ileal digestible lysine/kg dry matter, ‘Insecto Speen’, Coppens Diervoeding, Helmond, The Netherlands).
Litter size was standardized by cross-fostering within 3 days of age, resulting in an average number of 12.7 ± 0.2 piglets per litter at d4. The thirty-seven sows and their litters were allotted to one of four treatments (piglet feeding at d4 after birth and sow feeding at d10 post-partum) based on sow body weight and P2 back fat thickness at parturition and piglet birthdate and body weight at d0 and d4. Litter size at weaning did not differ between treatments (on average 12.4 ± 0.2 piglets per litter at d24).
Animals, housing and management
Pregnant primiparous TN70 sows (Norwegian Landrace x Large White, Topigs Norsvin, Vught, The Netherlands), inseminated with Tempo boar semen, were divided over two farrowing rooms (balanced for treatments) in three consecutive batches. The sows originated from one conventional farm and were housed at research facility Carus (Wageningen University & Research, The Netherlands) from two weeks before farrowing onwards. The pen was equipped with a farrowing area (2.85 × 1.80 m) and a free-movement area (1.85 × 1.80 m). The farrowing area was equipped with a crate (2.85 × 0.60 m) including a feed trough, a drinking nipple, a jute sack (around farrowing), a long-stemmed straw dispenser (from d10 post-partum) and three chew objects that alternated two times a week (metal chain with either one of three attachments: bolts, ball or PVC pipe) for the sow. The farrowing area included a drinking nipple, chew object (metal chain with bolts) and heating lamp for the piglets. The floor consisted of slats and rubber mats (2.00 × 1.75 m, in farrowing area) that served as nest for the piglets and provided lying comfort to the sow. The free-movement area was equipped with a drinking nipple for the sow. The sows were loose housed, except from shortly before farrowing until the first four days post-partum to minimize piglet crushing. During this initial period the free-movement area was not accessible to sows and piglets. Within 24 hours after birth, piglets received an ear tag and intramuscular iron injection and their birth weight and sex were determined. No teeth clipping, tail docking or castration were performed. In the farrowing rooms lights and radio were on from 6:00 to 19:30 h and lights were dimmed during the night. The room temperature was 23 °C around farrowing and gradually decreased to 20 °C at weaning.
At weaning (24.3 ± 0.1 days of age) a subset of 144 piglets (n = 9 weaner pens per treatment) was relocated in two weaner rooms (balanced for treatments) in two batches. Piglets were mixed with conspecifics from the same pre-weaning treatment and housed in pens (2.76 × 1.20 m) with four piglets (two males and two females) from three litters. Piglets were selected based on their sex and their body weight at d22 (close to the average weight of the litter and treatment group). Piglets with a history of medication and leg/claw problems were excluded from selection. The weaning weight of the selected piglets was 5.82 ± 0.13 kg, 6.06 ± 0.05 kg, 6.66 ± 0.04 kg and 6.30 ± 0.06 kg for RES-CON, RES-PL, FF-CON and FF-PL respectively. All weaner pens were identical and equipped with a conventional feed trough (three feeding spaces), drinking trough and chew object (metal chain with bolts). The floor was party slatted and partly covered with a rubber mat (1.75 × 1.20 m) that provided lying comfort to the piglets and prevented spillage of feed through the slats. Piglets were fed the nursery diet ad libitum. In the weaner rooms lights and radio were on from 7:00 to 19:00 h and room temperature gradually decreased from 25 to 23 °C at two weeks post-weaning, when the experiment ended and piglets were 39 days of age.
Measurements
Piglet growth
Piglets were individually weighed at d0 (within 24 h after birth), 4 (before commencing piglet feeding), 10 (before commencing feeding strategy of the sow), 17, 22, 24 (at weaning) before weaning and d1, 2, 5 and 15 post-weaning (at end of experiment).
Piglet behaviours
Piglets were marked using dark permanent hair dye (pre-weaning, Syoss professional performance permanent coloration, 1-1 noir) and animal marking spray (post-weaning, MS marking spray, MS Schippers, Bladel, The Netherlands). Feed-related behaviours in the farrowing pens were observed live at d9, 16 and 23 by 2-min instantaneous scan sampling for six sessions of one hour per day (i.e. 180 scans/piglet/d) using pen and paper. Sniffing, touching with snout or rooting the feed were defined as ‘exploring feed’ and eating or chewing feed was defined as ‘eating feed’. Sniffing, touching with snout, rooting or chewing on (toys of) the feeder, pushing or lifting toys of the feeder or shaking head while having toy of feeder in mouth were classified as ‘exploring feeder’. Feeder exploration and object play with (toys of the) the feeder are grouped as one behaviour in our study, as object exploration is often hard to distinguish from and precedes object play (reviewed by34). ‘Suckling’ was recorded on litter level as drinking milk from teat of sow (soft suckling noises). Observations were used to calculate time spent on feed-related behaviours, the proportion of piglets visiting the feeder (piglets that were scored either exploring the feed, exploring the feeder or eating feed at least once) and the proportion of piglets eating (piglets scored eating feed at least once). Behaviours in the weaner pens were recorded live on 30 and 39 days of age (6 and 14 days post-weaning respectively) by 2-min instantaneous scan sampling for six one-hour sessions per day using a Psion hand-held computer with the Pocket Observer 3.1 software package (Noldus Information Technology, Wageningen, The Netherlands). The ethogram with behaviours of interest in the weaner rooms is listed in Supplementary Table S4. Observation sessions started at 8:00, 9:15 and 10:30 h in the morning and at 14:00, 15:15 and 16:30 h in the afternoon.
Creep feed eaters and feed intake
Rectal swabs were taken at d10, 17, 22 and 24 to assess the presence of blue colour (Indigo carmine) and thereby to determine qualitative intake of creep feed (yes or no) on piglet level. Blue colour was not detected on the swabs at d10 and therefore this time point was excluded from analyses. Piglets were subsequently classified into different eater classes after Collins et al.38. Piglets of which blue colour was present on the swab on three measurement days were classified as ‘good/early eaters’. ‘Moderate eaters’ had blue colour on the swab on two measurement days and ‘bad eaters’ on only one measurement day. This may, by definition, not necessarily concern the last measurement day(s) and therefore these piglets cannot be called ‘late eaters’. Swabs of ‘non-eaters’ did not include blue colour at any of the measurement days. Pre-weaning feed intake was determined per litter between d10-17, d17-22 and d22-24 and post-weaning feed intake was determined per pen between 0-4h , 4-24h, d1-2, d2-5 and d5-15 post-weaning.
Body lesions and damage on piglets
We monitored the number of body lesions on the piglets at 4 hours and d1, d2 and d15 post-weaning (as a measure of aggression, according to Turner et al.39) and classified bite injuries on ears and tails of the piglets into no damage, bite marks or small wound at d15 post-weaning (as a measure of oral manipulation, according to van Nieuwamerongen et al.40).
Faecal consistency scores of piglets
Faeces in the weaner pens were scored daily for consistency according to Pedersen and Toft41. Score 1 (firm and shaped) and 2 (soft and shaped) represent normal faeces, and were combined into one score prior to data analysis. Score 3 (loose) and 4 (watery) represent diarrhoea. The highest faecal consistency score that was observed in a pen was selected on each measurement day and averaged over two weeks post-weaning to calculate the mean faecal consistency score (FCS) per pen. Faeces were removed on a daily basis after scoring to guarantee consistency scoring of fresh faeces.
Statistical analyses
Data processing
Piglet behaviours in the home pen were averaged per piglet per day and expressed as proportions of time. Eating feed was very low at d9 and exploring feed was very low at all ages before weaning (<0.1% of observation time) and were therefore excluded from analysis. After weaning, exploring feed, feeder and drinking trough were pooled into ‘exploring feed(er) and drinker’. Nosing, rooting and chewing the environment as well as chewing air were combined into ‘exploring environment’. The behavioural element ‘chewing poo’ was excluded from analyses because it was seen very rarely (0.01% of observation time). Lying with eyes closed and lying with eyes open were pooled into ‘inactive behaviour’. Standing and walking were pooled into ‘standing and walking’. Playing individually, socially and with chew object were summed and presented as ‘playing’. To investigate ‘nosing pen mates’, nosing body and snout contact were merged. The ear of the piglets with the highest damage score (either the left or right ear) was used in the analysis of ear damage. The number of body lesions on the piglets at weaning was subtracted from the number of body lesions at 4 hours after weaning.
Data analyses
Data were analysed with mixed models in the statistical software SAS 9.4 (SAS Institute Inc., Cary, NC, USA). Proportions of time spent on the different behaviours, the percentage of piglets per litter visiting the feeder and eating, the percentage of eaters per litter identified by rectal swab, and uniformity in BW (expressed as coefficient of variation) were analysed using a generalised linear mixed model (GLIMMIX) with a binomial distribution, logit link function and an additional multiplicative overdispersion parameter. The model did not converge with nosing environment at 2 weeks post-weaning as response variable, therefore this variable was analysed in a linear mixed model (MIXED). The number of body lesions and the number days with post-weaning diarrhoea were analysed using a GLIMMIX with a Poisson distribution, log link function and an additional multiplicative overdispersion parameter. The model did not converge with the total number of body lesions at 2 weeks post-weaning as response variable and was therefore analysed in a MIXED after square root transformation. Tail damage of weaner piglets was analysed in a GLIMMIX with a multinomial distribution and a cumulative logit link function. Data on the prevalence of watery diarrhoea in weaner pens were expressed as binary data and analysed with a GLIMMIX with a logit link and binary distribution. Individual creep feed classification of suckling piglets and ear damage on weaner piglets were analysed in a Fisher’s exact test, because there was an empty sub-classification category for the interaction effects. Continuous variables creep feed intake, average daily feed intake (ADFI), average daily gain (ADG), body weight (BW), feed conversion ratio (FCR) and mean faecal consistency score (FCS) were analysed in a MIXED procedure. For feed intake and ADG, totals over the pre- (d10–24) and post-weaning period (d0–15 post-weaning) were analysed, as well as effects on separate periods. Model residuals of the MIXED procedure were checked for normality. If model residuals were not normally distributed, data were transformed before analyses.
The model included the fixed effects of sow feeding (RES vs. FF), piglet feeding (PL vs. CON), their interactions, as well as batch (batch 1, 2 or 3). In the analysis of tail damage on weaner piglets, batch was excluded from the multinomial model (not significant). The number of body lesions at 4, 24 and 48 h post-weaning were analysed in a repeated GLIMMIX, therefore also including day and its interactions with the treatment groups as fixed effect. The number of body lesions at 2 weeks post-weaning was analysed separately in a GLIMMIX. Post-weaning ADFI and ADG were analysed in a repeated GLIMMIX and the four periods were also analysed separately. The same results were obtained and the results of the separate analyses are reported to facilitate interpretation. In addition, for behaviour, BW, ADG and the number of body lesions, the model included a random pen effect, nested within treatments and batch (farrowing pen for pre-weaning measurements and weaner pen for post-weaning measurements). Significant fixed effects were further analysed using differences of least squares means. Correlations were calculated at litter level using Pearson’s (normally distributed variables) and Spearman’s correlation coefficients (not normally distributed variables). Data are presented as (untransformed) means ± SEM based on pen averages. Differences at P < 0.05 were considered statistically significant and differences at 0.05 ≤ P < 0.10 were considered a trend.
Results
Feed-related behaviour and percentage of eaters before weaning
The play-feeder increased the time spent exploring the feeder by 3.5 to 6 times, irrespective of feed intake of the sow (PL vs. CON, d9: 0.68 ± 0.16 vs. 0.15 ± 0.04%; d16: 1.87 ± 0.32 vs. 0.54 ± 0.11%; d23: 2.18 ± 0.34 vs. 0.35 ± 0.05%; Fig. 2a). The treatments did not affect time spent eating creep feed at d16 (Fig. 2b), however RES-piglets doubled their time spent eating compared to FF-piglets at d23 (RES: 2.23 ± 0.55 vs. FF: 0.94 ± 0.26%). Time spent exploring the feeder correlated with time spent eating (r = 0.57; P < 0.0001) at all observation days (d9: r = 0.46, P < 0.01; d16: r = 0.51, P = 0.001; d23: r = 0.37, P = 0.02). Irrespective of feed intake of the sow and creep feed presentation method, the percentage of piglets within the litter visiting the feeder was 40, 68 and 83% at d9, 16 and 23 and the percentage observed to be eating was 2, 22 and 60% respectively. The play-feeder stimulated more piglets within a litter to visit the feeder (PL vs. CON, d9: 56.8 ± 5.9 vs. 22.3 ± 4.3%; d16: 80.6 ± 5.4 vs. 55.6 ± 7.3%; d23: 94.5 ± 2.4 vs. 70.4 ± 6.7%; Fig. 2c), but not to eat (Fig. 2d).
The percentage of creep feed eaters per litter, as identified by blue coloured swabs, was generally low up to d17, but increased with age (d10: 0%, d17: 5%, d22: 31%, d24: 37%). SF, PF and their interaction did not affect the percentage of eaters at d17 and 22. At weaning, litters of RES-sows included double as much eaters than litters of FF-sows (48.6 ± 8.6 vs. 26.5 ± 6.5% eaters/litter; Fig. 3a). The number of eaters per litter based on observations (seen eating at least once per observation day) correlated with the number of eaters per litter determined by the rectal swabs (blue colour on the swab) that were taken the day after (r = 0.71, P < 0.0001). Maternal feed restriction stimulated the number of piglets in better eater classes (P < 0.01) and the effect was more pronounced in litters that had access to a play-feeder, as shown in Fig. 3b (SF effect within PL: P < 0.0001, particularly moderate and good eaters, and SF effect within CON: P = 0.07, particularly bad eaters). The play-feeder also increased the number of piglets in better eater classes (P < 0.01, particularly the proportion of moderate and good eaters), but only within RES-piglets (PF effect within RES: P < 0.0001 and no effect within FF: P = 0.78).
Creep feed intake
Creep feed intake was generally low (especially up to d17 with on average ≤8 g/piglet) with on average 62 g/piglet between d4–24, and variable between litters, with total intake ranging from 3 to 399 g/piglet. As feed intake could be measured on litter level only, this was calculated as the intake per litter divided by the number of piglets in the litter and therefore the feed intake of individual piglets could be higher than 399 g. About 50% of the total feed intake was consumed during the last two days prior to weaning. Treatments did not affect feed intake between d17–22, but RES-piglets consumed more feed the last two days before weaning than FF-piglets (49 ± 13.9 vs. 15 ± 4.3 g/piglet; Table 1). Overall from the start of maternal feed restriction until weaning, RES-piglets tended to eat double the amount of feed compared to FF-piglets (d10–24: 81 ± 22.6 vs. 41 ± 10.7 g/piglet). Time spent exploring the feeder correlated with feed intake (r = 0.46; P < 0.0001). Analyses per day showed that time spent exploring the feeder did correlate with feed intake at d9 (r = 0.45, P < 0.01) and d16 (r = 0.31, P = 0.05), but not at d23 (r = 0.07, P = 0.66). Furthermore, time spent eating feed strongly correlated with feed intake (r = 0.83, P < 0.0001).
Piglet growth, body weight and litter uniformity in body weight before weaning
RES-piglets gained less weight between d10–17 (147 ± 5.3 vs. 188 ± 5.3 g/d), between d17–24 (209 ± 6.0 vs. 262 ± 7.4 g/d) and overall between d10–24 (213 ± 6.3 vs. 254 ± 6.7 g/d) than FF-piglets (Table 1). Consequently, RES-piglets tended to weigh less at d17 (4.47 ± 0.14 vs. 4.89 ± 0.22 kg; P = 0.09) and weighed less at weaning compared to FF-piglets (5.92 ± 0.16 vs. 6.72 ± 0.26 kg; Table 1). PL-piglets did not differ in ADG during lactation or BW at weaning from CON-piglets. SF, PF and their interaction did not affect homogeneity in BW within litters (CV) from d4 to weaning (Table 1).
Piglet behaviour after weaning
Ingestive behaviour
PL-piglets spent more time eating at week 2 after weaning compared to CON-piglets (PL: 11.8 ± 0.5 vs. CON: 10.4 ± 0.4%; Table 2). Moreover, PL-piglets showed more drinking than CON-piglets at week 1 (PL: 1.0 ± 0.1 vs. CON: 0.7 ± 0.1%) and week 2 post-weaning (PL: 1.2 ± 0.1 vs. CON: 0.9 ± 0.1%). Drinking was lower for RES-piglets compared to FF-piglets at week 2 post-weaning (RES: 0.8 ± 0.1 vs. FF: 1.2 ± 0.1%).
Exploratory behaviour
RES-piglets had a higher level of exploration towards the feed(er) and drinker compared to FF-piglets at week 1 post-weaning (RES: 2.8 ± 0.3 vs. FF: 2.0 ± 0.2%), but tended to have a lower level of exploration towards the feed(er) and drinker at week 2 post-weaning (RES: 2.1 ± 0.2 vs. FF: 2.6 ± 0.2%). Moreover, RES-piglets spent more time on exploring the environment at week 1 post-weaning compared to FF-piglets (RES: 24.1 ± 1.5 vs. FF: 18.1 ± 1.2%). PL-piglets tended to explore the feed(er) and drinker more at week 2 post-weaning in comparison with CON-piglets (PL: 2.6 ± 0.1 vs. CON: 2.1 ± 0.2%).
Postures and locomotion
RES-piglets tended to show less inactive behaviour compared to FF-piglets at week 1 post-weaning (RES: 42.9 ± 2.3 vs. FF: 48.7 ± 2.3%). The play-feeder did not affect (in)active behaviour after weaning.
Play behaviour
Playing was not affected by treatments.
Pig-directed behaviour
Ear biting was lower for RES-piglets compared to FF-piglets at week 2 post-weaning (RES: 0.4 ± 0.05 vs. FF: 0.7 ± 0.10%). PL-piglets tended to have higher levels of belly nosing compared to CON-piglets at week 1 post-weaning (PL: 0.5 ± 0.2 vs. CON: 0.2 ± 0.1%). The interaction between SF and PF affected aggression at week 1 post-weaning (P = 0.04), with less time spent on aggression in FF-CON piglets compared to FF-PL (P < 0.01), RES-CON (P < 0.05) and RES-PL piglets (P < 0.05). No effects were found on nosing pen mates, manipulating pen mates, tail biting and mounting.
Other behaviour
No interaction and main effects of SF and PF were found for the other behaviours after weaning (comfort behaviour: 0.42 ± 0.03%; eliminating: 0.68 ± 0.03%; data not shown).
Body lesions and damage on piglets after weaning
Body lesions in the first two days post-weaning
The number of fresh body lesions was high the first day after weaning, but decreased thereafter with 16.8 ± 1.9, 22.2 ± 2.6 and 11.0 ± 1.8 lesions at 4, 24 and 48 hours post-weaning respectively. Interactions between SF x PF were found on the number of body lesions throughout the first two days after weaning (P < 0.02; no interaction with time). RES-piglets had less body lesions than FF-piglets, but only within CON (P < 0.01; RES-CON: 10.6 ± 2.2 vs. FF-CON: 23.7 ± 4.8 lesions, with intermediate levels of RES-PL: 10.8 ± 1.8 and FF-PL: 11.1 ± 1.8 lesions).
Body lesions and damage at week 2 post-weaning
On average 4.0 ± 0.4 lesions were found on the body of weaned piglets at two weeks after weaning. PL-piglets had a lower number of body lesions (3.1 ± 0.5 vs. 4.8 ± 0.6 lesions; Table 3). Moreover, there were less PL-piglets with wounds on their ears than CON-piglets (P = 0.02), but only when reared by FF-sows (PF effect within FF: P = 0.01; Fig. 4a). The percentage of piglets with tail damage was affected by the interaction between SF × PF (P = 0.02) and tended to be affected by the play-feeder (P = 0.05; Fig. 4b). RES-PL had less piglets with tail damage than RES-CON (P < 0.01, OR = 0.15, 95% CI = 0.04–0.61) and tended to have less piglets with tail damage than FF-PL (P = 0.06, OR = 0.26, 95% CI = 0.06–1.05). FF-CON and RES-CON (P = 0.20, OR = 1.96, 95% CI = 0.70–5.50) as well as FF-CON and FF-PL (P = 0.78, OR = 1.17, 95% CI = 0.40–3.44) did not differ in the proportion of piglets with tail damage.
Feed intake, growth and faecal consistency after weaning
Maternal feed restriction and the play-feeder increased feed intake in the first four hours after weaning (RES: 24 ± 4 vs. FF: 17 ± 3 g/piglet, PL: 24 ± 4 vs. CON: 17 ± 3 g/piglet; P < 0.05 for both). The treatments tended to interact in their effect on feed intake in this period (P = 0.08), showing that RES-PL had a higher intake of feed within the first four hours after weaning compared to the other three treatment groups (RES-CON: 17 ± 3, RES-PL: 31 ± 6, FF-CON: 16 ± 4, FF-PL: 18 ± 4 g/piglet; P ≤ 0.01 for comparisons). Maternal feed restriction and the play-feeder during lactation increased the ADFI (Fig. 5a) and ADG (Fig. 5b) after weaning, with the effect of the play-feeder lasting until the end of the experiment. Maternal feed restriction improved the intake of feed on the first day (RES: 101 ± 11 vs. FF: 32 ± 7 g/day), the second day (RES: 210 ± 10 vs. FF: 114 ± 15 g/day) and between d2–5 post-weaning (RES: 282 ± 9 vs. FF: 237 ± 15 g/day; Fig. 5a). The play-feeder improved the daily intake of feed on the second day (PL: 188 ± 14 vs. CON: 136 ± 18 g/day), between d2–5 (PL: 286 ± 10 vs. CON: 232 ± 13 g/day), between d5–15 (PL: 405 ± 14 vs. CON: 360 ± 15 g/day) and during the two weeks after weaning (PL: 345 ± 11 vs. CON: 300 ± 12 g/day; Table 3).
Interactions between SF × PF were found for ADG on the first and second day after weaning (Fig. 5b). Maternal feed restriction improved ADG between d0–2 post-weaning (RES: 128 ± 17 vs. FF: −32 ± 21 g/day; P < 0.0001) and tended to improve ADG over the two weeks after weaning (RES: 283 ± 9 vs. FF: 263 ± 9 g/day; Table 3). RES-piglets did not differ anymore in BW from FF-piglets at d15 post-weaning (RES: 10.18 ± 0.17 vs. FF: 10.43 ± 0.14 kg; Table 3). The play-feeder improved ADG between d0–2 (PL: 91 ± 24 vs. CON: 6 ± 27 g/day; P = 0.001), d2–5 (PL: 262 ± 12 vs. CON: 222 ± 12 g/day), d5–15 (PL: 342 ± 10 vs. CON: 313 ± 7 g/day; Fig. 5b) and during the two weeks after weaning (PL: 293 ± 8 vs. CON: 253 ± 7 g/day; Table 3). Consequently, PL-piglets had a higher weight than CON-piglets at d15 post-weaning (PL: 10.57 ± 0.13 vs. CON: 10.04 ± 0.16 kg; Table 3). The treatments did not affect FCR (Table 3). The play-feeder resulted in a shorter duration of diarrhoea (PL: 1.72 ± 0.37 vs. CON: 3.00 ± 0.47 days of diarrhoea during 2 weeks post-weaning), a lower FCS (PL: 0.13 ± 0.03 vs. CON: 0.26 ± 0.04) and a lower prevalence of watery diarrhoea in the weaner pens in the first two weeks post-weaning (PL: 16.7 vs. CON: 61.1% of pens; Table 3). SF did not affect the faecal consistency parameters after weaning.
Discussion
A low lactational feeding level of the sow was used to reduce the energy intake of piglets from milk and to investigate whether creep feed intake is homeostatically driven, while a play-feeder was used to present creep feed in an explorative and playful context and to study whether creep feed consumption is exploratory driven. Maternal feed restriction motivated piglets to eat creep feed and thereby eased the weaning transition, as reflected by a higher feed intake and growth and less body lesions, mainly in the first two days after weaning. Although the play-feeder did not improve creep feed intake of piglets, it stimulated feeder exploration before weaning and had a broad and long-term beneficial effect on ingestive behaviours, feed intake, growth, faecal consistency and body lesions and damage in the two weeks after weaning.
Consistent with predictions, maternal feed restriction by 50% resulted in a 16% lower ADG of piglets during lactation and a 12% lower weaning weight. Previous studies showed that a low lactational feeding level of the sow reduced energy supply from milk, due to a lower milk production and changes in milk composition
Comparison and Evaluation of Four Species of Macro-Algaes as Dietary Ingredients in Litopenaeus vannamei Under Normal Rearing and WSSV Challenge Conditions: Effect on Growth, Immune Response, and Intestinal Microbiota.
Results
Trial 1
Growth Performance
As is shown in Table Table4,4, the survival rate was in the scope of 99–100% among all treatments with no significant differences (P > 0.05). Growth performance of shrimp fed the D5 diet was significantly higher than that of shrimp fed the control and D4 diets (P < 0.05) but without statistically significant difference with other diets (P > 0.05). FBW of shrimp in D5 diet was significantly higher than that of other groups, except D3 group (P > 0.05). FCR of shrimp fed the control diet was significantly higher than that of shrimp fed D2, D3, and D5 diets (P < 0.05) but without significant difference with shrimp fed D4 diet (P > 0.05), while PER showed the inverse trend with FCR.
Table 4
Effect of five experimental diets on biological performance of L. vannamei juveniles.
Items | IBW/g | FBW/g | WG/% | SGR/(%/d) | Survival/% | FCR | PER |
---|---|---|---|---|---|---|---|
D1 | 0.66 ± 0.01 | 6.19 ± 0.04a | 841 ± 8.07a | 4.00 ± 0.02a | 100 ± 0.00 | 1.03 ± 0.01c | 2.42 ± 0.03a |
D2 | 0.65 ± 0.01 | 6.35 ± 0.06a | 882 ± 2.56abc | 4.08 ± 0.01bc | 99.17 ± 0.83 | 0.96 ± 0.01ab | 2.60 ± 0.02b |
D3 | 0.66 ± 0.01 | 6.63 ± 0.06b | 913 ± 11.94bc | 4.13 ± 0.02bc | 100 ± 0.00 | 0.92 ± 0.02a | 2.73 ± 0.04c |
D4 | 0.64 ± 0.01 | 6.16 ± 0.09a | 869 ± 11.70ab | 4.06 ± 0.02ab | 99.17 ± 0.83 | 0.99 ± 0.01bc | 2.50 ± 0.06ab |
D5 | 0.65 ± 0.02 | 6.58 ± 0.07b | 921 ± 23.73c | 4.15 ± 0.04c | 99.17 ± 0.83 | 0.92 ± 0.01a | 2.73 ± 0.02c |
Whole Body Composition
As can be seen in Table Table5,5, the moisture content in control diet was significantly higher than other diets (P < 0.05). D5 diet had the highest protein content and was significantly higher than that of shrimp fed other diets (P < 0.05). The lipid content of shrimp in D4 was significantly higher than that in D1, D3, and D5 (P < 0.05) but without significant difference with shrimp fed D2 diet (P > 0.05). The ash content of shrimp fed the control diet was significantly lower than that of shrimp fed the other four macro-algaes diets (P < 0.05); however, no significant difference was found in ash content among the four macro-algaes diets treatments (P > 0.05).
Table 5
Effect of five experimental diets on whole body composition (%) of L. vannamei juveniles.
Items | D1 | D2 | D3 | D4 | D5 |
---|---|---|---|---|---|
Whole body | |||||
Moisture | 75.91 ± 0.03d | 75.25 ± 0.03b | 75.15 ± 0.03a | 75.39 ± 0.04c | 75.28 ± 0.01b |
Protein | 72.46 ± 0.03a | 73.60 ± 0.01c | 73.69 ± 0.04c | 73.29 ± 0.04b | 73.80 ± 0.04d |
Lipid | 5.92 ± 0.04a | 6.59 ± 0.04cd | 6.45 ± 0.07bc | 6.66 ± 0.10d | 6.38 ± 0.03b |
Ash | 13.81 ± 0.03a | 14.30 ± 0.15b | 14.32 ± 0.03b | 14.30 ± 0.11b | 14.34 ± 0.05b |
Apparent Digestibility Coefficiency
Apparent digestibility coefficiencts of dry matter from the D2, D3, and D5 treatments were higher than that from the control and D4 treatments (P < 0.05) (Table (Table6).6). ADC of protein from the D5 treatment showed the highest value and was significantly higher than that from the control, D2, and D4 treatment (P < 0.05) while with no significant difference with the D3 treatment (P > 0.05). ADC of lipid from the D4 treatment was significantly lower than that from the other treatments (P < 0.05), but there is no statistical significant difference with the other four treatments (P > 0.05).
Table 6
ADCs (%) of L. vannamei juveniles in five experimental diets.
Items | D1 | D2 | D3 | D4 | D5 |
---|---|---|---|---|---|
Digestibility | |||||
Dry matter | 78.67 ± 0.33a | 85.67 ± 0.67c | 85.33 ± 0.67c | 82.33 ± 0.33b | 85.67 ± 0.33c |
Protein | 79.67 ± 0.88a | 83.67 ± 0.33b | 84.67 ± 0.33bc | 80.33 ± 0.33a | 85.67 ± 0.33c |
Lipid | 97.33 ± 0.33b | 97.67 ± 0.33b | 97.33 ± 0.88b | 91.67 ± 0.88a | 97.67 ± 0.33b |
Immune Response
There was no significant differences in SOD, MDA, and carbonyl protein contents activity among all diets treatments (P > 0.05) in trail 1 (Table (Table7).7). While TAS and ProPO activities of shrimp fed the macroalgae-containing diets were significantly higher than those of shrimp fed the control diet (P < 0.05), and no significant differences were found in TAS and PO activities among shrimp fed the four macroalgae-containing diets (P > 0.05).
Table 7
Effect of experimental diets on immune response (TAS, μmol g-1 organ; SOD, U mg-1 protein; PO, O.D.490 nm; MDA, nmol mg-1 protein; carbonyl protein, nmol mg-1 protein) of L. vannamei both during the 8 weeks rearing period and after the WSSV injection challenge test.
Diets | Trial1: During the 60-day rearing period | Trial 2: After the challenge test | ||||||||
---|---|---|---|---|---|---|---|---|---|---|
D1 | D2 | D3 | D4 | D5 | D1 | D2 | D3 | D4 | D5 | |
Hepatopancreas antioxidant parameters | ||||||||||
TAS | 2.34 ± 0.04 aB | 4.20 ± 0.03 bB | 4.22 ± 0.05 bB | 4.21 ± 0.02 bB | 4.20 ± 0.05 bB | 1.97 ± 0.04 aA | 3.18 ± 0.02 cA | 3.19 ± 0.01 cA | 2.55 ± 0.05 bA | 3.21 ± 0.03 cA |
SOD | 2.27 ± 0.04 A | 2.29 ± 0.09 A | 2.32 ± 0.04 A | 2.29 ± 0.07 A | 2.30 ± 0.02 A | 4.22 ± 0.03 cB | 3.14 ± 0.02 aB | 3.15 ± 0.04 aB | 3.34 ± 0.06 bB | 3.14 ± 0.02 aB |
PO | 5.16 ± 0.04 aA | 6.78 ± 0.06 bA | 6.82 ± 0.07 bA | 6.79 ± 0.05 bA | 6.80 ± 0.05 bA | 7.38 ± 0.07 aB | 10.26 ± 0.04 cB | 10.24 ± 0.04 cB | 9.61 ± 0.08 bB | 10.21 ± 0.18 cB |
Hepatopancreas oxidative injury parameters | ||||||||||
MDA | 12.33 ± 0.09 A | 12.27 ± 0.10 A | 12.26 ± 0.07 A | 12.26 ± 0.05 A | 12.26 ± 0.04 A | 32.26 ± 1.08 bB | 21.54 ± 0.47 aB | 21.89 ± 0.23 aB | 21.97 ± 0.44 aB | 22.55 ± 0.23 aB |
Carbonyl | 1.22 ± 0.02 A | 1.19 ± 0.05 A | 1.21 ± 0.04 A | 1.22 ± 0.03 A | 1.19 ± 0.04 A | 2.90 ± 0.04 cB | 1.70 ± 0.03 aB | 1.71 ± 0.04 aB | 2.19 ± 0.02 bB | 1.69 ± 0.03 aB |
protein |
What’s New in the Performance Diet Pro 2001 v3.0.6 serial key or number?
Screen Shot
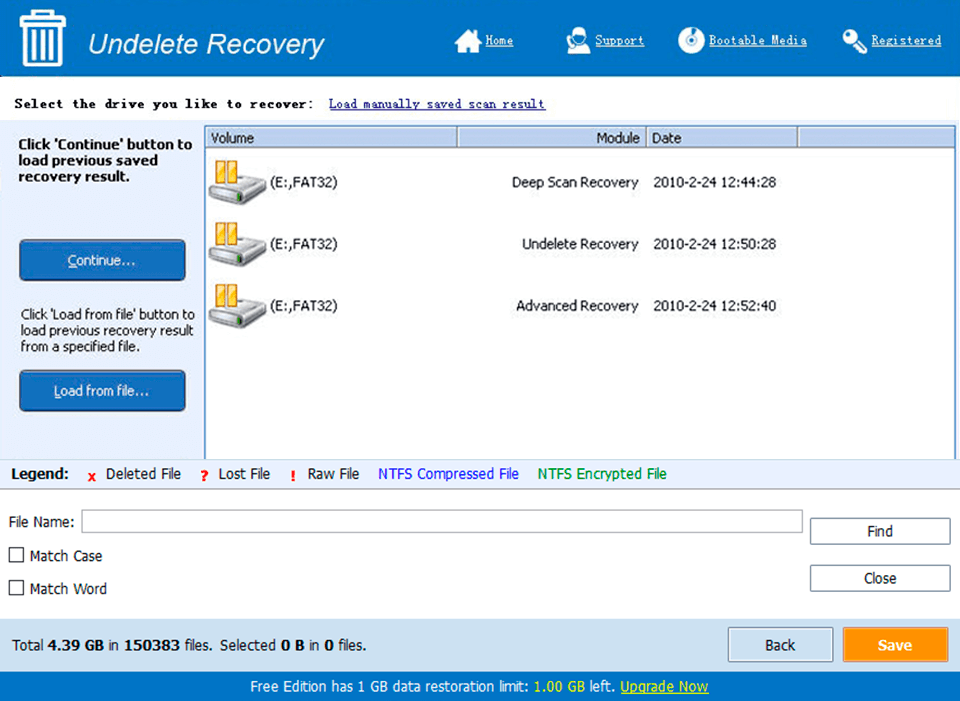
System Requirements for Performance Diet Pro 2001 v3.0.6 serial key or number
- First, download the Performance Diet Pro 2001 v3.0.6 serial key or number
-
You can download its setup from given links: